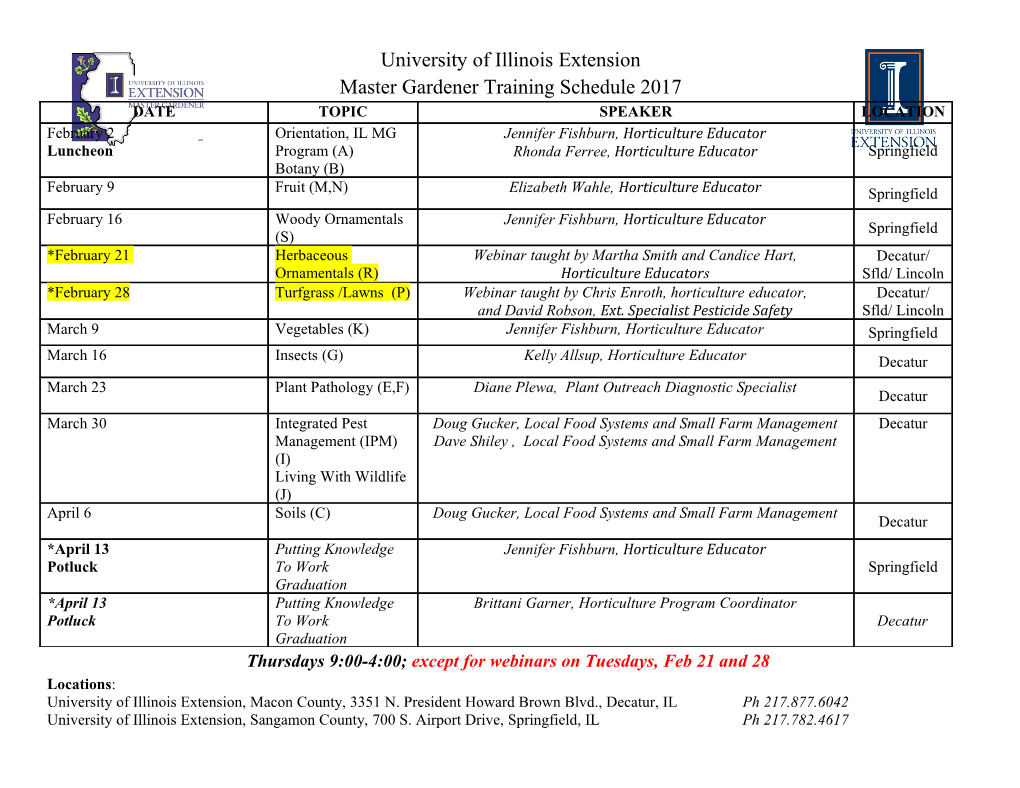
J Mater Sci (2015) 50:2849–2858 DOI 10.1007/s10853-015-8847-x Enhanced antimicrobial activity with faceted silver nanostructures Mauricio Rojas-Andrade • Adam T. Cho • Peiguang Hu • Shannon J. Lee • Christopher P. Deming • Samantha W. Sweeney • Chad Saltikov • Shaowei Chen Received: 1 December 2014 / Accepted: 7 January 2015 / Published online: 24 January 2015 Ó Springer Science+Business Media New York 2015 Abstract Faceted silver nanostructures including trian- of metal nanostructures in the manipulation of their anti- gular nanoprisms, nanotetrahedra, and nanodecahedra were microbial activity. synthesized via a facile photochemical method at con- trolled wavelengths using spherical nanoparticles as the seeds. Scanning transmission electron microscopy studies showed that the resulting nanostructures were much larger Introduction in size (20–50 nm) than the spherical seed nanoparticles (under 5 nm), and X-ray diffraction as well as high-reso- The biocidal effects of silver have been known for thou- lution transmission electron microscopy measurements sands of years as there is evidence of ancient Greeks confirmed that these nanostructures exhibited predomi- incorporating silver into wound dressings to stimulate nantly {111} faceted surfaces. Importantly, the silver healing and storing water in silver vessels for preservation nanostructures demonstrated markedly better antimicrobial [1]. The medical uses of silver have since expanded, as the activity than the spherical seed nanoparticles as evidenced first scientific publication on its effectiveness for treating by a lower minimum inhibitory concentration and more newborn postpartum eye infections opened many scien- dramatic changes in both growth rate and lag phase at tists’ curiosity and led to a surge of research into the lower concentrations, which were attributed to the greater applications of silver as a biocidal agent [2]. Silver has reactivity of the {111} faceted surfaces toward oxygen-rich been found to be the most promising bactericidal agent in a bacterial surface moieties that allowed for more rapid plethora of scenarios, attributed to its exceptional activity localization to bacterial cells and increased interactions at relatively low concentrations and limited toxicity toward with structurally vital outer-membrane proteins. These eukaryotic cells [3]. Its effectiveness stems from its broad- results highlight the significance of surface morphologies spectrum effect on bacterial cells, as silver ions interact with multiple cellular targets affording the biggest advan- tage over target-specific, small-molecule antibiotics. The mechanisms of silver toxicity have been thoroughly Electronic supplementary material The online version of this investigated by several groups, and there is general con- article (doi:10.1007/s10853-015-8847-x) contains supplementary material, which is available to authorized users. sensus on three primary mechanisms that lead to cell death: membrane lysis via peroxidation of structural lipids by M. Rojas-Andrade Á A. T. Cho Á P. Hu Á reactive oxygen species (ROS), protein inactivation result- & S. J. Lee Á C. P. Deming Á S. W. Sweeney Á S. Chen ( ) ing from the binding and oxidation of thiol moieties on Department of Chemistry and Biochemistry, University of California Santa Cruz, California 95064, USA structurally relevant residues, and transcriptional arrest due e-mail: [email protected] to DNA condensation caused by the binding of silver ions to DNA molecules [4]. For instance, Kim et al. have shown & C. Saltikov ( ) evidence for ROS generation by silver ions through electron Department of Microbiology and Environmental Toxicology, University of California Santa Cruz, California 95064, USA paramagnetic resonance (EPR) studies and membrane lysis e-mail: [email protected] of affected cells by transmission electron microscopy 123 2850 J Mater Sci (2015) 50:2849–2858 (TEM), lending evidence for silver’s role as an initiator of molecular oxygen and hydroxide molecules as evidenced membrane peroxidation [5]. In the same study, the role of by cyclic voltammetry studies comparing the dissolution free radicals as active species was also supported as bacterial between different silver facets [21]. Given the evidence, cultures having the antioxidant N-acetylcysteine added in nanostructures with predominantly {111} faceted surfaces addition to silver nanoparticles were found to have much warrant further investigation to shed light on the nature of higher survivability. Silver, as a soft acid, reacts preferen- their enhanced antimicrobial activity. This is the primary tially with soft bases such as thiols which are essential motivation of the present study. components of protein tertiary structures. It has been shown Photochemical synthesis of silver nanoprisms has gained that these soft–soft interactions are the underlying driving much attention since Jin et al. first reported a simple light- force behind silver’s toxicity toward bacterial cells, as a induced ripening of silver nanospheres into nanoprisms [22]. study conducted by Xu et al. in 2012 comparing the toxicities This process was found to produce triangular, hexagonal, and of metal ions with varying softness found a trend in which circular nanoplates which were nearly flat (\10 nm thick) in softer metals such as silver, mercury, and cadmium dem- high yield. The growth of these anisotropic nanostructures is onstrated higher antimicrobial activity than harder metals the result of excitation of nanoparticle surface plasmon res- such as zinc, nickel, and cobalt [6]. Several other groups have onance (SPR) causing redox processes to occur preferen- studied interactions of silver with thiol moieties of cysteine tially at surfaces with more intense induced electromagnetic residues on proteins critical for cellular respiration, such as fields. Specifically, when the in-plane dipole resonance is NADH dehydrogenase, which is responsible for causing excited, ‘‘hot’’ electrons and ‘‘hot’’ holes are generated and cellular transcriptional arrest [7–10]. Additionally, silver has concentrated at surfaces on the nanoparticle in the same been found to react preferentially with N7 of the GC and AT plane as the dipole excitation. At these surfaces, ‘‘hot’’ holes base pairs of DNA and not the phosphate groups of the DNA oxidize surface-bound citrate molecules into 1,3-aceton- backbone as previously thought, resulting in helix conden- edicarboxylate and carbon dioxide though the photo-Kolbe sation and transcriptional arrest [11]. Due to the multitude of mechanism, where the hydroxyl group of sodium citrate mechanisms by which silver elicits its antimicrobial activity, donates an electron pair to the central carbon atom forming a it has become the most promising candidate for antimicrobial carbon–oxygen double bond which subsequently causes the applications such as surface coatings, medical wound central carbon to undergo heterolytic cleavage with the rest dressings, and water filtration. of the citrate molecule thereby releasing carbon dioxide [23]. With the advent of nanotechnology, highly effective NMR studies have previously shown supporting evidence of silver nanostructures have now been developed which have emerging 1,3-acetonedicarboxylate, with a corresponding a markedly enhanced activity when compared to silver salts peak at d = 3.49 ppm, upon photoexcitation of the silver [5, 12–15]. Recent advancements have been made in nanospheres into triangular nanoprisms [24]. Localization of delivery mechanisms for biocidal silver, with hydrogels, hot holes onto surface-bound citrate molecules initiates these polymers, and porous structures such as zeolites being uti- irreversible decarboxylation reactions which transfer elec- lized for both structural support and release of silver ions trons to the silver nanoparticle causing in-plane surfaces to [16–18]. This improved activity is largely attributed to sil- charge cathodically. This subsequently reduces Ag? ions in ver nanostructures having an exceptionally large surface- solution to Ag0 resulting in selective in-plane growth [23– area-to-volume ratio with smaller particles having a larger 25]. Triangular nanoprisms are found to have a maximum- degree of exposed surface area. This enhanced active sur- induced electromagnetic field localized at the tips from face area affords a greater level of contact with bacterial cell electron energy loss spectroscopy (EELS) when their in- walls and allows for increased rates of silver ion dissolution plane SPR is excited. This allows for these photo-induced thereby resulting in higher bactericidal activity [19]. Pal redox cycles to occur most rapidly on these surfaces, which et al. recently found evidence that bactericidal activity is explains why these structures dominate the final particle also shape-dependent, with triangular silver nanoprisms population after prolonged irradiation. This has indeed been having predominantly {111} facets demonstrating superior observed experimentally [26]. Such a plasmon-mediated activity when compared to spherical and cubical nanopar- growth method provides a unique control of the structural ticles of similar size [20]. This study indicates that the morphology through synthetic parameters such as pH, silver activity is highly dependent on surface structure and sug- ion concentration, citrate concentration, and most interest- gests that the silver {111} facet is most favorable for anti- ingly, excitation wavelength [27–29]. Triangular nanop- microbial applications due to its high atom density.
Details
-
File Typepdf
-
Upload Time-
-
Content LanguagesEnglish
-
Upload UserAnonymous/Not logged-in
-
File Pages10 Page
-
File Size-