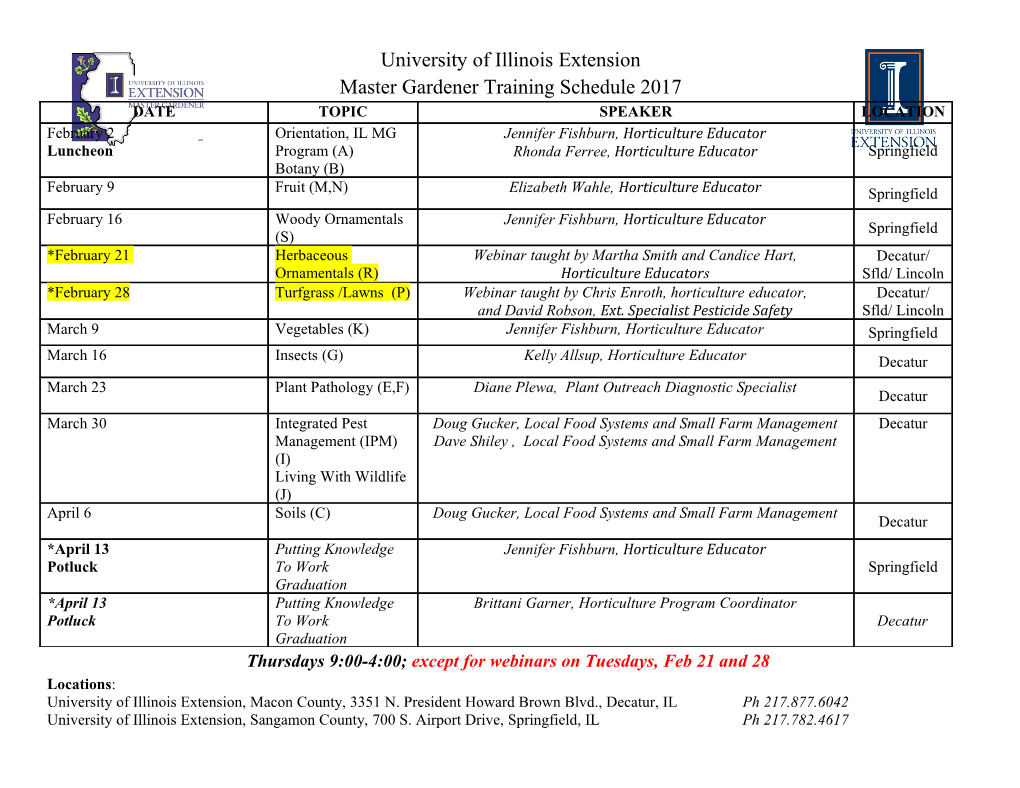
Markus Haase Lectures on Functional Calculus { 21st International Internet Seminar { November 29, 2017 CAU Press 2017 Contents 1 Holomorphic Functions of Bounded Operators ............ 7 1.1 Polynomial Functional Calculus . .7 1.2 Power Series Functional Calculi . .8 1.3 The Dunford{Riesz Calculus . 10 1.4 From Bounded to Unbounded Operators . 18 1.5 Supplement: Functional Calculus for Matrices . 19 Exercises . 22 2 Unbounded Operators and Abstract Functional Calculus . 25 2.1 Unbounded Operators. 25 2.2 Multiplication Operators . 27 2.3 Functional Calculus for Multiplication Operators . 29 2.4 Abstract Functional Calculus (I) | Definition . 30 2.5 Abstract Functional Calculus (II) | Generators . 34 Exercises . 36 3 Borel Functional Calculus ................................ 39 3.1 Normal Operators on Hilbert Spaces . 39 3.2 Measurable Functional Calculus . 41 3.3 Projection-Valued Measures . 43 3.4 Borel Functional Calculus . 46 3.5 Supplement: Commutation Results . 49 Exercises . 51 4 The Spectral Theorem .................................... 55 4.1 Three Versions of the Spectral Theorem . 55 4.2 Proof: Bounded Self-Adjoint Operators . 58 4.3 Proof: From Continuous Functions to Multiplication Operators 59 4.4 Proof: From Bounded to Unbounded Operators . 62 4.5 Supplement: Normal Operators . 64 Exercises . 66 v vi Contents 5 Fourier Analysis .......................................... 69 5.1 Strongly Continuous Semigroup Representations . 69 5.2 The Regular Representations. 71 5.3 Averaging a Representation and the Convolution of Measures 73 5.4 The Fourier Transform . 77 Exercises . 81 6 Integral Transform Functional Calculi .................... 85 6.1 The Fourier{Stieltjes Calculus . 85 6.2 Bounded C0-Semigroups and the Hille{Phillips Calculus . 88 6.3 General C0-Semigroups and C0-Groups . 92 6.4 Supplement: Continuity Properties and Uniqueness . 96 6.5 The Heat Semigroup on Rd .............................. 99 6.6 Supplement: Subordinate Semigroups . 103 Exercises . 106 Appendix .....................................................1001 A.1 Notational and Terminological Conventions . 1001 A.2 Calculus in Banach Spaces . 1005 A.3 Vector-valued Holomorphic Functions . 1010 A.4 Unbounded Operators. 1014 A.5 Operators on Hilbert Space . 1024 A.6 The Bochner Integral . 1029 A.7 Complex Measures. 1034 Chapter 1 Holomorphic Functions of Bounded Operators 1.1 Polynomial Functional Calculus Let X be a Banach space (over C) and A 2 L(X) a bounded operator on it. Then for every polynomial 2 n p = a0 + a1z + a2z + ··· + anz 2 C[z] (1:1) we can form the (likewise bounded) operator 2 n p(A) := a0 + a1A + a2A + ··· + anA 2 L(X): The space of polynomials C[z] is a unital algebra and the mapping Ψ : C[z] !L(X); p 7! Ψ(p) := p(A) is a (unital) algebra homomorphism. In other words, Ψ is a representation of the unital algebra C[z] on the vector space X by bounded operators. Obviously, any representation Ψ of C[z] on X by bounded operators is of the form above: simply define A := Ψ(z) and find Ψ(p) = p(A) for all p 2 C[z]. One may call Ψ a calculus since it reduces calculations with operators to calculations with other (here: formal) objects. Strictly speaking, however, this calculus is not (yet) a functional calculus, since a polynomial is not a function. It can become a function when it is interpreted as one, but this needs the specification of a domain, say D ⊆ C. Let us denote, for a polynomial p 2 C[z], its interpretation as a function on D by pjD. Then the indeterminate, which we have called \z" here, is mapped to the function zjD : D ! C; z 7! z: Here and in all what follows, we shall denote this function by z. If p has the form given in (1.1) then 7 8 1 Holomorphic Functions of Bounded Operators 2 n pjD = a0 + a1z + a2z + ··· + anz : Let us take D = C, which is somehow the most natural choice. Then the mapping p 7! pjC is injective. Hence we can take its inverse and compose it with Ψ from above to obtain a unital algebra homomorphism Φ : fpolynomial functions on Cg ! L(X);Φ(pjC) = Ψ(p): Now this is indeed a functional calculus, called the polynomial functional calculus. Note that A = Φ(z) and Φ is uniquely determined by this value. 1.2 Power Series Functional Calculi So far, the property that A is bounded was not used at all. Everything was pure algebra. Taking boundedness into account we can extend the polynomial functional calculus to a functional calculus of entire functions. Namely, each entire function f 2 Hol(C) has a unique power series representation 1 2 n X n f = a0 + a1z + a2z + ··· + anz ··· = anz : n=0 The convergence is absolute and locally uniform, so that in particular 1 X n janj r < 1 for each r 2 R+. n=0 Hence, we can define 1 X n f(A) := Φ(f) := anA 2 L(X); n=0 the series being absolutely convergent in the Banach space L(X). Clearly, if f is a polynomial (function), this definition of f(A) agrees with the previous. The so obtained mapping Φ : Hol(C) !L(X); f 7! f(A) is a homomorphism of unital algebras, because the usual rules of manipulation with absolutely convergent series are the same in C and in L(X) (actually in all Banach algebras). Notably, the multiplicativity of Φ follows from the fact that the Cauchy product formula 1.2 Power Series Functional Calculi 9 1 1 1 n X X XX an bn = ajbn−j n=0 n=0 n=0 j=0 for absolutely convergent series holds in C as well as in L(X). The functional calculus for entire functions works for every bounded operator A. If we take kAk or even all the norms kAnk, n 2 N, into account, we can extend the calculus further, for instance as follows: n −n Fix r > 0 such that M(A; r) := supn≥0 kA kr < 1. (One can take r = kAk, but in general r < kAk is possible.) Abbreviate Dr := B(0; r) (and 1 D = D1), and denote by A+(Dr) the set of functions f : Dr ! C that can be represented as a power series 1 X n f(z) = anz (jzj ≤ r) (1:2) n=0 such that 1 X n kfk 1 := kfk 1 := janj r < 1: (1:3) A+ A+(Dr ) n=0 1 Note that each f 2 A+(Dr) is holomorphic on Dr and continuous on Dr = B[0; r]. In particular, the coefficients an are uniquely determined by the function f, so kfk 1 is well defined. Using the Cauchy product formula, A+ 1 it is easy to see that A+(Dr) is a unital Banach algebra. (Exercise 1.2). 1 For f 2 A+(Dr) with representation (1.2) we can now define 1 X n f(A) := Φ(f) := anA : n=0 The condition (1.3) ensures that this power series is absolutely convergent and computations as before show that 1 Φ :A+(Dr) !L(X); f 7! f(A) is a homomorphism of unital algebras. Moreover, one has the norm estimate 1 kf(A)k ≤ M(A; r) kfk 1 (f 2 A ( r)) A+ + D as is easily seen. Remark 1.1. The case r = 1 is particularly important. An operator with M(A; 1) < 1 is called power bounded. So, power bounded operators have 1 a (bounded) A+(D)-calculus, a fact that will become important later in this course. 10 1 Holomorphic Functions of Bounded Operators Note that in the above construction one has A = Φ(z), and by this condi- tion Φ is uniquely determined as a continuous unital algebra homomorphism 1 A+(Dr) !L(X). 1.3 The Dunford{Riesz Calculus The above power series functional calculi for an operator A use only informa- tion about the asymptotic behaviour of the norms kAnk. In this section we shall construct a holomorphic functional calculus for A that is based purely on the location of its spectrum. Review of elementary spectral theory Recall from an elementary functional analysis course that the spectrum of A 2 L(X) is the set s(A) := fλ 2 C : λ − A is not invertibleg: (We write λ − A instead of λI − A.) The complement r(A) := C n s(A), an open subset of C, is called the resolvent set of A. The mapping R(·;A): r(A) !L(X);R(λ, A) := (λ − A)−1 is the resolvent. It satisfies the resolvent identity R(λ, A) − R(µ, A) = (µ − λ)R(λ, A)R(µ, A)(λ, µ 2 r(A)) (1:4) and is holomorphic. (See Appendix A.3 for an introduction to vector-valued holomorphic mappings.) P1 −(n+1) n A number λ 6= 0 belongs to r(A) whenever the series n=0 λ A converges, in which case this series equals R(λ, A). (This follows from the Neumann series theorem.) In particular, λ 2 r(A) if jλj > kAk and then 1 kR(λ, A)k ≤ : jλj − kAk Hence, the spectrum is compact and not empty (by Liouville's theorem), except in the case X = f0g. The spectral radius r(A) := supfjλj : λ 2 s(A)g satisfies r(A) ≤ kAk. 1.3 The Dunford{Riesz Calculus 11 Dunford{Riesz{Calculus, \baby" version We start with a \baby" version of the Dunford{Riesz calculus and take s > r(A), i.e., s(A) ⊆ Ds. For a function f 2 Hol(Ds) define 1 Z Φ(f) := f(z)R(z; A) dz (1:5) 2pi γr where 0 < r < s is such that still s(A) ⊆ Dr and γr is the contour which traverses the boundary of Dr once in counterclockwise direction.
Details
-
File Typepdf
-
Upload Time-
-
Content LanguagesEnglish
-
Upload UserAnonymous/Not logged-in
-
File Pages151 Page
-
File Size-