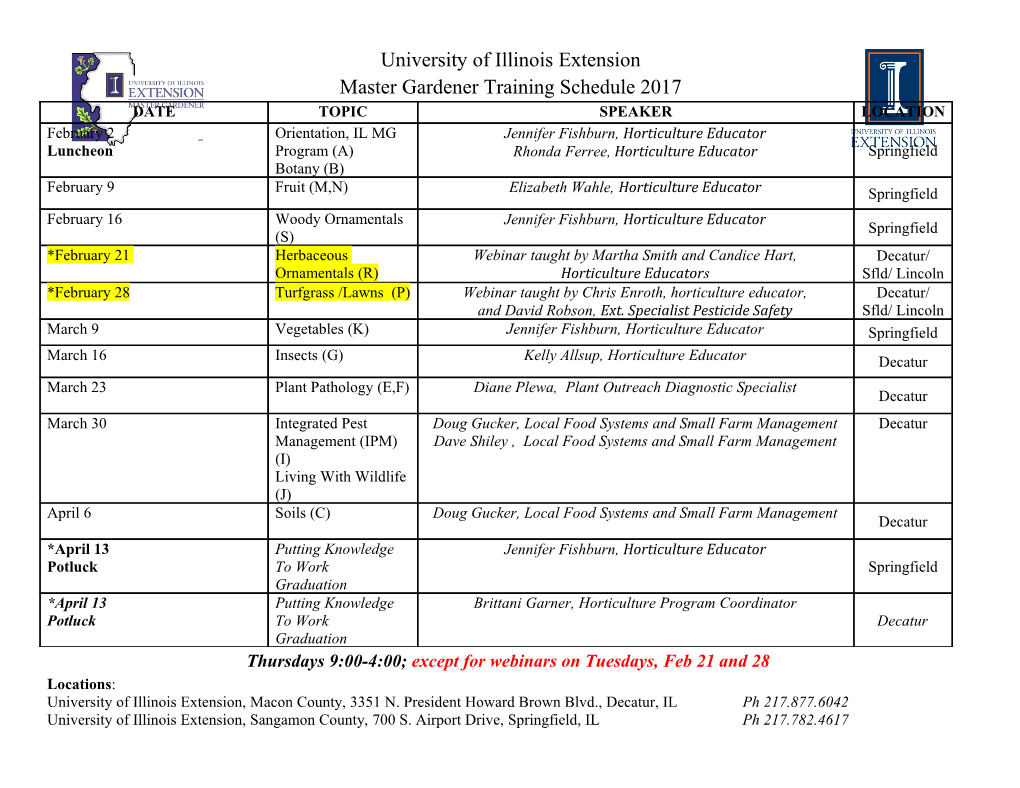
Electrochemical Conversion of Nitrogen Trifluoride as a Gas-to-Solid Cathode in Li Batteries The MIT Faculty has made this article openly available. Please share how this access benefits you. Your story matters. Citation He, Mingfu et al. "Electrochemical Conversion of Nitrogen Trifluoride as a Gas-to-Solid Cathode in Li Batteries." Journal of Physical Chemistry Letters 9, 16 (July 2018): 4700-4706 © 2018 American Chemical Society As Published http://dx.doi.org/10.1021/acs.jpclett.8b01897 Publisher American Chemical Society (ACS) Version Author's final manuscript Citable link https://hdl.handle.net/1721.1/121525 Terms of Use Article is made available in accordance with the publisher's policy and may be subject to US copyright law. Please refer to the publisher's site for terms of use. Electrochemical Conversion of Nitrogen Trifluoride as a Gas-to-Solid Cathode in Li Batteries Mingfu He†,Yuanda Li†, Rui Guo†, and Betar M. Gallant†,* †Department of Mechanical Engineering, Massachusetts Institute of Technology, MA 02139, United States *E-mail: [email protected] 1 ABSTRACT: Nonaqueous metal-gas batteries have emerged as a growing family of primary and rechargeable batteries with high capacities and energy densities. We herein report a high- capacity primary Li-gas battery that uses a perfluorinated gas, nitrogen trifluoride (NF3), as the cathode reactant. Gravimetric capacities of ~1100 and 4000 mAh/gC are achieved at 25 and + 55°C, respectively (at 20 mA/gC), with discharge voltages up to 2.6 V vs Li/Li . NF3 reduction - occurs by a 3e /NF3 process, yielding polycrystalline lithium fluoride (LiF) on a carbon cathode. The detailed electrochemical NF3 conversion mechanism is proposed and supported by solid- and liquid-phase characterization and theoretical computation, revealing the origin of observed discharge overpotentials and elucidating the significant contribution of N-F bond cleavage. These findings indicate the value of exploring fluorinated gas cathodes for primary batteries; moreover, they open new avenues for future targeted electrocatalyst design and cathode materials synthesis applications benefitting from conformal coatings of LiF. TOC GRAPHIC 2 Nonaqueous metal-gas batteries have undergone intensive development over the past decade. Though metal-gas batteries have a long history dating back to early primary Li-sulfur dioxide 1 2 (Li-SO2) and Li-oxygen (Li-O2) batteries, the field experienced a resurgence recently based on 3 renewed enthusiasm for the prospective rechargeability of Li-O2 batteries. With high theoretical 4,5 energy densities (~3500 Wh/kg) compared with Li-ion batteries (~250 Wh/kg), Li-O2 batteries are attractive for electric vehicles if suitable reversibility and cycle life can be achieved. Unfortunately, their viability is still in doubt owing to persistent challenges, including parasitic side reactions, high charge overpotentials and poor cycle life.5 Regardless, attempts to address these challenges have led to vital new insights and needed innovation in electrochemical technologies. This is evidenced strongly in the recent efforts to 6 7 8 expand the metal-gas family; past years have seen the emergence of the Na-O2, K-O2, Li-CO2, 9 10 11 Li-O2/CO2, Na-CO2, and rechargeable Li-SO2 battery. While most efforts to date have focused on modifying the metal anode for O2 batteries, there is significant opportunity to explore novel chemistries for gas cathodes, specifically those that offer prospects of high capacity and voltage. As many gas cathodes are not necessarily rechargeable at the outset, near-term applications include high-energy density primary batteries for remote and backup power, autonomous underwater vehicles, and military and space applications. However, such investigations can contribute essential new understanding to electrochemical conversion mechanisms of gases and also help to pave the way forward towards longer-term development of rechargeable systems. Our own recent efforts have shown that gas cathode reactions need not be limited to common O2, CO2 or SO2, but can also involve nominally ‗inert‘, highly fluorinated gases.12 3 The present work explores the viability of a highly-fluorinated gas, nitrogen trifluoride (NF3), as a gas cathode in a Li battery. NF3 is a colorless, nonflammable gas with routine usage in the 13 microelectronics industry. When paired with a Li anode, NF3 can theoretically undergo a three- electron reduction, yielding stoichiometric LiF and N2 gas. This reaction requires two NF3 - + molecules, viz. 2 NF3 + 6 e + 6 Li = 6 LiF + N2, and N-N bond formation. Given the stability of the products, LiF and N2(g), the 6-electron reaction has a highly negative Gibbs free energy of reaction, ΔrG°, of -3351 kJ/mol, and corresponds to a remarkable theoretical electrochemical potential of 5.79 V vs. Li/Li. Moreover, the theoretical discharge capacity, normalized to the weight of reactants, is 876 mAh/g. If this free energy can be harnessed in a battery, a theoretical -1 gravimetric energy density of 5970 Wh kg can be achieved. However, NF3 is generally considered chemically inert, as reactions usually need to be initiated by high energy,14–16 and to the best of our knowledge, its electrochemical activity has not been investigated. We thus herein studied the use of NF3 as the reactive gas in a Li battery employing a glyme- based electrolyte and a catalyst-free carbon cathode (Scheme 1). First, to determine the suitability of using NF3 in a nonaqueous Li environment, the electrochemical behavior was screened in typical battery electrolytes consisting of 0.07 - 0.1 M lithium perchlorate (LiClO4; concentration adjusted in accordance with solubility) in diethylene glycol diethyl ether (diglyme), tetraethylene glycol dimethyl ether (TEGDME), acetonitrile, propylene carbonate (PC), and dimethyl sulfoxide (DMSO). Swagelok-type Li-NF3 cells (Figure S1) were constructed within an argon glovebox using a PC electrolyte-stabilized Li metal foil as the anode and Vulcan Carbon (VC) as the cathode. In acetonitrile only, due to its reactivity with Li, LiFePO4 was used as the anode.17 Additional details can be found in Supporting Information (SI). Galvanostatic discharges at 100 mA/gC are shown in Figure S2. While varying degrees of activity were 4 a Scheme 1. Schematic of a primary Li-NF3 battery. + a. Li, Li -containing nonaqueous electrolyte, and carbon/dissolved NF3(g) are used as the anode, electrolyte, and cathode, respectively. During discharge, two NF3 molecules are nominally - reduced to form LiF solids and liberate N2(g) by an overall six-electron process, 2NF3 + 6e + + 6Li 6LiF + N2. observed in all solvents, the discharge profile in acetonitrile exhibited sloping behavior and did not exhibit a clear end of discharge within the tested voltage window. Meanwhile, the capacities in PC and DMSO were relatively low (< 300 mAh/gC); furthermore, the separator using DMSO turned brown after discharge, indicating electrolyte decomposition. In contrast, discharge capacities in glyme-based solvents yielded moderate capacities of 460 - 500 mAh/gC with evident voltage quasi-plateaus around 2.10 - 2.18 V vs. Li/Li+; thus, diglyme was selected as the solvent for continued analysis. Physicochemical properties of the NF3-LiClO4 (diglyme) system were next investigated in detail. The solubility of NF3 in H2O was previously reported to be as low as 0.8 mM in H2O at the partial pressure of 14.70 psi (1 atm) and 298 K,18 but solubilities in organic solvents have not been widely reported. Our attempts to measure NF3 solubility in diglyme using a method adapted from that reported previously12 were challenged due to low solubilities. We did, however, verify 19 the presence of NF3 in the electrolyte from F-NMR measurement (Figure S3) and estimated its 5 solubility, given instrument resolution, to be below 1 mM. Although this solubility is lower than those typically observed in a metal-gas battery (e.g., 1 - 10 mM for O2 in nonaqueous electrolytes), it did not impede electrochemical measurement, as discussed further below. The chemical compatibility of NF3 with electrolyte components was also investigated. NF3 is an oxidizer; although highly electronegative fluorides reduce the nucleophilicity of the nitrogen lone pair, parasitic reactivity of NF3 with ether oxygens or salt anions was a possible concern. 1 Thus, H NMR and Raman measurements were conducted on electrolytes bubbled with NF3 and 1 maintained for 24 hours under an NF3 headspace. As shown in Figure S4, H NMR peaks at = 3.38, 3.56, and 3.65 ppm are assigned to diglyme, and the peak positions and integral ratios (3:2:2, respectively) remained identical after NF3 treatment, indicating that no reaction occurred. The electrolyte stability is further corroborated by identical Raman spectra (Figure S5) before and after NF3 dissolution. Galvanostatic discharge behavior of Li-NF3 batteries was next investigated. As shown in Figure 1a, upon discharge at low currents (20 mA/gC, 25 °C), the voltage decreased from OCV and reached a quasi-plateau of 2.10 - 2.25 V, which was retained throughout discharge to a + capacity of 950 mAh/gC before the voltage decreased to the cutoff of 1.6 V vs. Li/Li . The eventual observed ―sudden death‖19 of the battery discharge is likely due to the accumulation of electrically insulating products on the cathode, mainly composed of polycrystalline LiF, as discussed later. We note that OCVs of the Li-NF3 battery during the discharge, obtained from the galvanostatic intermittent titration technique (GITT) measurement, were significantly higher than the discharge voltage and also decreased steadily from 2.98 – 2.84 V (Figure S6a and b), likely due to gradual coating of the carbon by these discharge products. Further increasing the current density from 20 mA/gC to 500 mA/gC under direct galvanostatic conditions led to a decrease in 6 Figure 1. Galvanostatic discharge profiles of Li-NF3 batteries. (a) Rate capability (20 – 500 mA/gC) at 25 °C; (b) Temperature effect (25 – 55 C) at 50 mA/gC; (c) Rate capability at 55°C; and (d) Comparison of attainable discharge capacities from panels (a) and (c).
Details
-
File Typepdf
-
Upload Time-
-
Content LanguagesEnglish
-
Upload UserAnonymous/Not logged-in
-
File Pages22 Page
-
File Size-