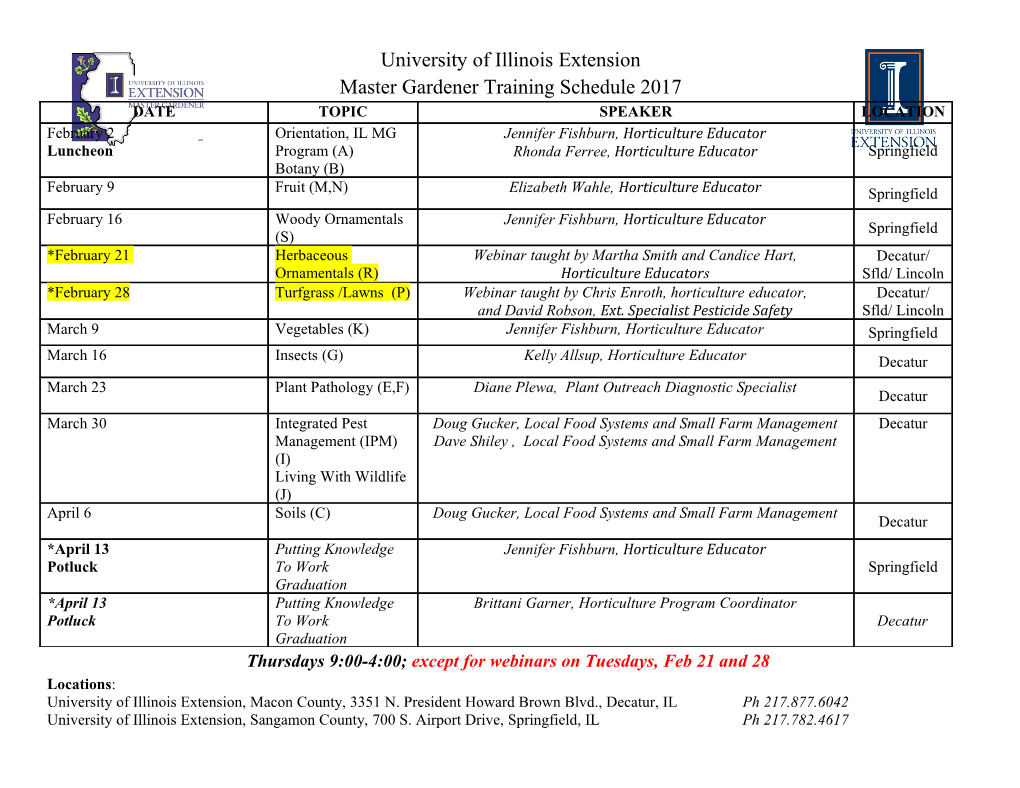
Max-Planck-Institut fur¨ Astrophysik Parametric Studies of Hydrodynamic Instabilities in the Supernova Core by Two- and Three-Dimensional Simulations Leonhard Scheck Vollst¨andiger Abdruck der von der Fakult¨at fur¨ Physik der Technischen Universit¨at Munchen¨ zur Erlangung des akademischen Grades eines Doktors der Naturwissenschaften genehmigten Dissertation. Vorsitzender: Univ.-Prof. Dr. Lothar Oberauer Prufer¨ der Dissertation: 1. Priv.-Doz. Dr. Hans-Thomas Janka 2. Univ.-Prof. Dr. Andrzej J. Buras Die Dissertation wurde am 05.09.2006 bei der Technischen Universit¨at Munchen¨ eingereicht und durch die Fakult¨at fur¨ Physik am 29.11.2006 angenommen. Contents 1 Introduction 5 1.1 The explosion mechanism . 6 1.1.1 Core collapse . 6 1.1.2 Prompt explosions . 7 1.1.3 Delayed explosions . 7 1.2 The anisotropy of core collapse supernovae . 8 1.2.1 Evidence for anisotropy . 8 1.2.2 Possible origins . 9 1.3 Neutron star kicks . 10 1.3.1 The neutron star velocity distribution . 10 1.3.2 Neutron star kick mechanisms . 11 1.4 Aims of this thesis . 12 2 Computational approach and numerical methods 15 2.1 Hydrodynamics and gravity . 15 2.2 Neutrino transport and neutrino source terms . 18 2.3 Numerical grid . 19 2.4 Boundary conditions . 21 2.4.1 Hydrodynamics . 21 2.4.2 Neutrinos . 21 2.4.3 Moving the inner boundary to a different mass shell . 23 3 Explosion anisotropy in two dimensions 25 3.1 Preliminaries . 25 3.1.1 Initial models and initial perturbations . 25 3.1.2 Overview of the simulations . 27 3.1.3 Definitions and approximations for post-processing the simulations . 28 3.2 Two representative models . 34 3.2.1 The character of the flow . 34 3.2.2 Acceleration and recoil of the neutron star . 37 3.2.3 Possible origin of the low-order modes . 40 3.2.4 Comparison with previous works . 41 3.2.5 Sensitivity to the seed perturbations . 44 3.3 Dependence on the initial model and the core luminosity . 47 3.4 The effects of rotation . 49 3.4.1 Evolution of the rotation rate . 50 3.4.2 Morphology . 50 3.4.3 Energetics . 54 3.4.4 Neutron star recoil . 54 3.5 Robustness and long-time evolution of the neutron star recoils . 55 3.5.1 Anisotropic neutrino emission . 55 3 4 Contents 3.5.2 Inertial mass of the neutron star . 56 3.5.3 Neutron star contraction and gravitational potential . 57 3.5.4 Long-time evolution of the neutron star kicks . 58 3.6 Implications for the neutron star velocity distribution . 59 4 The origin of the global anisotropy 63 4.1 Hydrodynamic instabilities . 64 4.1.1 Linear and non-linear convective growth of perturbations . 64 4.1.2 The advective-acoustic cycle . 65 4.2 Simulations . 67 4.2.1 A model without neutrinos . 67 4.2.2 Models with suppressed convection . 70 4.2.3 Models with typical explosion energies . 75 4.3 Interpretation of the linear phase . 76 4.3.1 Measuring oscillation period and cycle efficiency . 76 4.3.2 Identification of the instability mechanism . 79 4.4 Interpretation of the non-linear phase . 82 4.4.1 AAC triggers convection . 82 4.4.2 Explosion vs. non-explosion . 83 4.4.3 The AAC as an alternative to convection . 86 5 Three-dimensional effects 87 5.1 Model parameters . 88 5.1.1 Initial models and boundary conditions . 88 5.1.2 Numerical grid . 88 5.1.3 Additional 2D simulations . 89 5.2 Non-rotating models . 89 5.2.1 Evolution of global quantities . 89 5.2.2 Convective overturn . 94 5.2.3 Neutrino-driven outflows . 100 5.2.4 Global anisotropy and neutron star kicks . 104 5.3 A rotating model . 113 5.3.1 Missing polar downflows . 113 5.3.2 Anisotropic, neutrino-driven outflows . 116 5.3.3 Explosion anisotropy and downflow distribution . 118 5.3.4 Neutron star kick . 122 5.3.5 Spin-kick alignment? . 124 6 Summary and conclusions 127 A Neutrino transport 137 A.1 Transport equation . 137 A.2 Neutrino distribution function . 139 A.3 Optical depth . 140 A.4 Source terms . 142 B Explosion energy 149 C Hydrodynamics in an accelerated frame of reference 155 1 Introduction About two times per century a massive star dies in our Milky Way. Instead of passing away silently, these stars die in spectacular explosions | supernovae. In the first seconds of such an extremely violent event, more energy is set free than was radiated away by the star during its whole life. Only a small part of this energy is released in the form of electromagnetic radiation, but this amount already suffices for the explosion to outshine even its parent galaxy. For this reason supernovae have been noticed since the beginnings of astronomical observations. Reports of strange stars that appear for some time and vanish again can be found in records of Chinese astronomers more than 2000 years ago. Supernova observations appear also in early Korean, Japanese, Arabic and European records. Two of the first \modern" astronomers were fortunate to witness such rare1 events, Tycho Brahe in 1572 and Johannes Kepler in 1604. With the advent of modern astronomical instruments it became possible to study the spectra of supernovae. This allowed Minkowski (1941) to distinguish two classes of supernovae, a clas- sification that is still in use today: The spectra of Type II supernovae show strong Balmer hydrogen lines near maximum brightness, whereas the spectra of Type I supernovae show no signs of hydrogen. The absolute peak magnitude and the shape of the lightcurve also differ for the two classes. Differences in the spectra and the light curve behaviour within the two classes led to subclassifications. The early spectra of Type Ia supernova show lines of silicon, in contrast to types Ib and Ic, which do not. Type Ib and Ic supernovae differ in the presence or absence of helium lines, respectively. The lightcurve of Type II-L supernovae declines linearly after maximum light, whereas Type II-P supernova are characterised by a plateau phase in the light curve. This purely phenomenological classification does not involve any theoretical understanding of the underlying explosion mechanism that could explain the observed differences. A first hint that there are actually two fundamentally different mechanisms at work came from the identification of the environments, in which the supernovae occurred. Type Ia supernovae show no tendency to occur in a certain galaxy type, nor in a certain location within the galaxy. In contrast, Type II, Type Ib, and Type Ic supernovae are observed only in spiral galaxies, and most of them occur in regions with a high star formation rate, i.e., in spiral arms and H II regions. This points 1Only supernovae occurring in the Milky Way could be observed by early astronomers. Using modern instru- ments, nowadays more than 100 supernovae per year are detected in remote galaxies. 5 6 CHAPTER 1. INTRODUCTION to the possibility that the progenitors of Type Ia supernovae are old, low-mass stars and that supernovae of the other classes originate from younger, short-lived, massive stars. Currently the generally agreed upon interpretation is that Type Ia supernovae are thermonuclear explosions of white dwarfs, which do not leave any compact remnant behind. All other types are thought to arise from the collapse of a massive star's core, which triggers an explosion that disrupts the outer layer of the star and leads to the formation of a neutron star or even a black hole. The various subclasses differ in the presence of hydrogen and helium layers at the moment of explosion. The stars can have stripped these outer stellar layers by stellar wind loss or mass transfer in binary star systems. The processes taking place in the first seconds of such a \core collapse supernova", which are still not well-understood, are the subject this work. 1.1 The explosion mechanism In 1934, Baade & Zwicky realized that enormous amounts of gravitational binding energy must be released, when the core of an ordinary star collapses to a neutron star. They suggested that this energy powers the supernova explosion of the dying star. The existence of extremely compact neutron stars had been proposed by Landau only two years before (after the discovery of the neutron by Chadwick in 1932), but such objects were found only some decades later, when periodic radio signals were detected from pulsars, rotating neutron stars with strong magnetic fields. Yet, the process itself, i.e. the mechanism leading to the explosion and the formation of a neutron star, is still not completely understood after more than seventy years of research. 1.1.1 Core collapse There is, however, some agreement about the processes taking place right before the onset of the explosion (see Bethe 1990 for a more detailed description). Near the end of their lives, stars with main sequence masses of more than about 8 M have gone through several burning phases and have developed an onion-shell like structure. For main sequence masses larger than about 56 56 10 M the core of such a progenitor star consists of iron-group elements (mostly Fe and Ni), which are the most tightly bound elements and consequently cannot release further energy by nuclear burning. This core is surrounded by shells of lighter nuclei { around the iron core there is Si/S, and further out O/Ne/Mg, C/O, He, and finally H (the latter two shells may have been lost due to stellar winds or mass transfer in binary systems).
Details
-
File Typepdf
-
Upload Time-
-
Content LanguagesEnglish
-
Upload UserAnonymous/Not logged-in
-
File Pages159 Page
-
File Size-