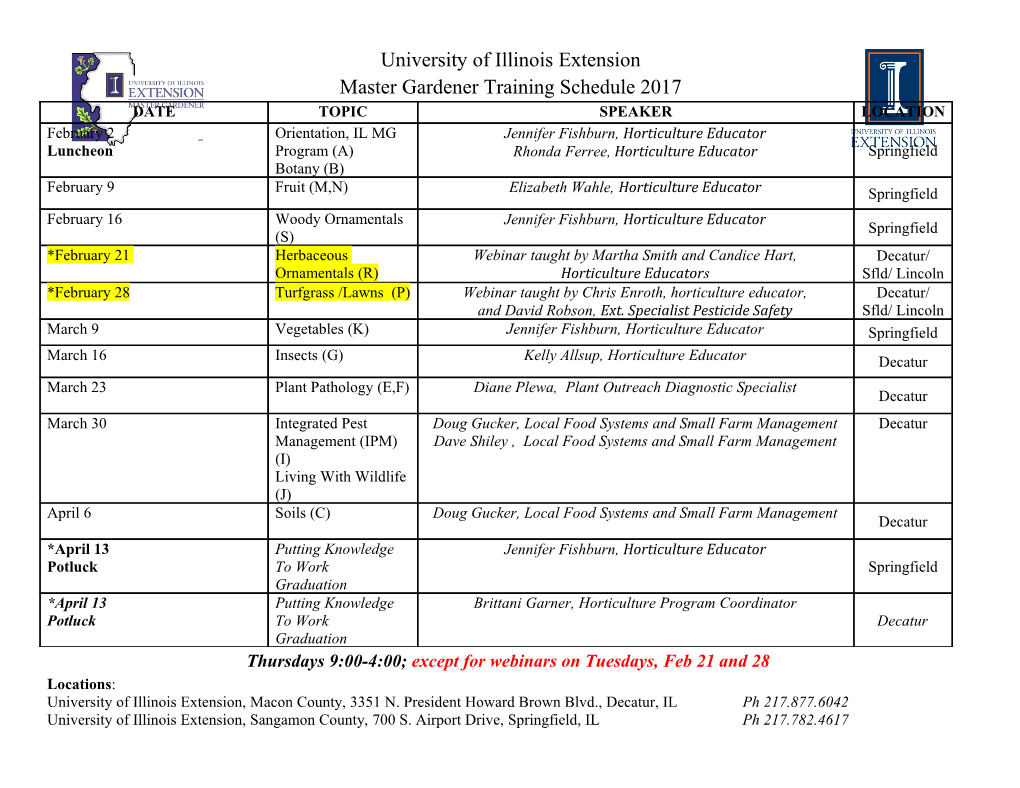
OPTIMIZATION OF 2-STROKE SI ENGINE CONCEPT WITH STOICHIOMETRIC MIXTURE Oldřich VÍTEK, Jan MACEK Czech Technical University, Prague Company Logo Outline 1. Introduction – motivation and goals 2. Engine Model – tools and calibration, idealization of some engine parameters 3. Computed Cases – constraints and definition of Parameters being compared 4. Results – initial results at 4-cyl. engines, final results at 3-cyl. engine 5. Conclusion 2 1. Introduction Motivation hHigh low end torque of two stroke engines. hModerate BMEP in comparison with a 4- stroke engine. hNatural EGR/Internal EGR (IGR) – support of CAI/HCCI at low loads. hExisting 4-stroke engine modified (advanced VVA necessary) to enable 2-stroke mode. The Three Way Catalyst applied to satisfy emission limits. 3 1. Introduction Goals hTo optimize ideal 2-stoke engine in terms of BSFC while satisfying prescribed constraints – steady operation only. hTo compare 2-stroke engine concept with 4- stroke one under the same/similar conditions for the case of passenger car engine. 4 1. Basic Engine Parameters 4-cylinder engine Bore-to-Stroke Ratio [-] 0.889 Relative Displ. Volume [%] 100 Compression Ratio [-] 10.2 Charging Charged Fuel Gasoline Configuration R4 No. of Intake Valves 2 (VVA) No. of Exhaust Valves 2 (VVA) 5 1. Basic Engine Constraints 4-cylinder engine Required Relative Power [%] 100 Air Excess [-] 1.0 Max. In-Cylinder [bar] 160 Pressure Max. Boost Pressure [bar] 6 Free Oxygen in Exhaust [%] 5 Max. IGR [%mass] no limit 6 1. Basic Engine Parameters 3-cylinder engine Bore-to-Stroke [-] 1.0 Relative Displ. Volume [%] 80 Compression Ratio [-] 9.8 Charging Charged Fuel Gasoline Configuration R3 No. of Intake Valves 2 (VVA) No. of Exhaust Valves 2 (VVA) 7 1. Basic Engine Constraints 3-cylinder engine Required Relative Power [%] 125 Air Excess [-] 1.0 Max. In-Cylinder [bar] 160 Pressure Max. Boost Pressure [bar] 4 Free Oxygen in Exhaust [%] 5 Max. IGR [%mass] 20 8 2. Engine Model – Software Tools hGT-Power code used (1-D approach to pipes, 0-D approach to other engine parts). hmodeFRONTIER for multi-variable single- objective optimization using genetic algorithm (MOGA-II; setting for 8 independent variables: 20 generations; DOE: SOBOL, 500 designs) 9 2. Engine Model hBase engine model – 4-cylinder 4-stroke calibrated engine model (experimental/geometrical data provided by manufacturer) hIdeal 2-stroke engine model derived from base engine model (4-stroke) => minor model changes and idealization of some parts (more details are presented later on) hIdeal 4-stroke model derived from ideal 2- stroke one (switching the operation mode back to 4-stroke) 10 2. Engine Model – Predictive Submodels hDetail FE based in-cylinder wall temperature solver hIn-cylinder heat transfer coefficient (calibrated Woschni formula) hFriction – calibrated Chen-Flynn formula (using either experimental 4-stroke engine data or detailed MS Excel friction model) hCombustion – based on ROHRs evaluated from experimental data (no knocking, no influence of engine load) 11 2. Engine Model (4-stroke) Calibration WOT 12 2. Engine Model (4-stroke) Calibration WOT 13 2. Engine Model (4-stroke) Calibration WOT – indicated pressure n=3000rpm 14 2. Engine Model (4-stroke) Calibration WOT – indicated pressure n=3000rpm 15 2. Engine Model – Idealization of a Compressor hMechanically driven compressor with variable gear (to control boost pressure) – isentropic efficiency 80% (constant within the whole operation domain), overall mechanical efficiency 96%. 16 2. Engine Model – Idealization of a Valvetrain hIdeal VVA valve train – quite fast open/close ramp, ideal valve lift profile (no dynamic effects), no collisions taken into account. hVVA will be found necessary for exhaust valve (intake valve might use VVT only). 17 2. Engine Model – Idealization of IC hIdeal intercooler – air outlet temperature is almost constant and rather low in the whole engine operating range. hPressure loss realistic for plain intercoolers. 18 2. Engine Model – Idealization of Combustion and Mixture Parameters hDirect gasoline injection is assumed (no danger of fuel occurrence in exhaust manifold due to scavenging). hNo knocking is taken into account => combustion is always positioned into its thermodynamic optimum regardless of engine load/speed hStochiometric mixture properties ( λ=1 ) for all engine operating points (to enable proper function of TWC) 19 3. Computed Cases hDirect comparison of a 2-stroke engine with a 4-stroke one (4-cylinder engine) under full load operation. hInfluence of compression ratio (4-cylinder engine). hInfluence of scavenging model (4-cylinder engine). hComplete engine map (steady operation) – estimation of NEDC fuel consumption to compare it with a real SI engine (3-cylinder 20 engine). 3. Computed Cases – Optimization hGenetic algorithm (MOGA II) has been applied to find optimum BSFC respecting constraints in any of further presentations hThe following variables were optimized: hValve open timing and duration for intake/exhaust valve (4 variables). hIntake/exhaust manifold length/diameter (4 variables) – for some cases, otherwise pipe geometry was fixed (no optimization needed). 21 3. Computed Cases - Constraints initial calculations of 4-cylinder engine Max. In-Cyl. Pressure [bar] 160 Max. Boost Pressure [bar] 6 Max. Total EGR [%] no limit Free Oxygen in Exhaust [%] 5 hBoth 4-cylinder crankshafts were designed in the same manner, i.e., planar crank (180° angle between webs – for the initial comparison only). h3-cylinder crankshafts have the same 240°/120° web angle configuration 22 3. Computed Cases - Constraints final calculations (3-cylinder engine only) Max. In-Cyl. Pressure [bar] 160 Max. Boost Pressure [bar] 4 Max. Total EGR [%] 20% Free Oxygen in Exhaust [%] 5 23 4. Results: Detailed info (4-cylinder) 2-stoke Engine; Full Load; 6000rpm; εεε=11: EO Inlet EC pressure IO Exhaust IC pressure Exhaust pressure 24 4. Results: Detailed info (4-cylinder) 4-stoke Engine; Full Load; 6000rpm; εεε=11: Inlet EC pressure EO IO Exhaust IC pressure Exhaust pressure 25 4. Results: Power (4-cylinder) WOT; Comparison with ideal 4-stroke eng.: 26 4. Results: Max. Pressure (4-cylinder) Full Load; Comparison with ideal 4-stroke eng.: ideal 4-stroke engine ideal 2-stroke engine CR 11.0 (λ=1) ideal 2-stroke engine CR 10.2 (λ=1) 27 4. Results: Free O2 - exh. (4-cylinder) Full Load; Comparison with ideal 4-stroke eng.: 28 4. Results: Rest Gas %, IC (4-cylinder) WOT; Comparison with ideal 4-stroke eng.: 29 4. Results: Boost Pressure (4-cylinder) WOT; Comparison with ideal 4-stroke eng.: 30 4. Results: Compressor Power WOT; Comparison with ideal 4-stroke eng.: 31 Results: Trapping Ratio (4-cylinder) WOT; Comparison with ideal 4-stroke eng.: 32 4. Results: b.s.f.c. (4-cylinder) WOT; comparison with ideal 4-stroke eng.: experiment – real 4- ideal 2-stroke engine stroke engine ( λ<1) CR 10.2 (λ=1) ideal 2-stroke engine CR 11.0 (λ=1) real 4-stroke engine ( λ=1) ideal 4-stroke engine 33 4. Definition of Energy Fluxes hThe more profound analysis of results requires to present energy fluxes during energy transformation. hThe following definition of normalized energy fluxes is applied in the following graphs: influence of additional 2 high-pressure strokes phase only (exhaust & (compression& intake) at a expansion 4stroke engine strokes) 34 4. Results: Heat Transfer Flux % WOT; 4 cylinder engine 35 4. Results: Enthalpy Flux % in Exhaust Gas; WOT; 4 cylinder engine 36 4. Results: Indicated Efficiency (total cycle); WOT; 4 cylinder engine ideal 2-stroke engine ideal 4-stroke better engine better 37 Results: Integral Data (4-cylinder) Full Load; Comparison with ideal 4-stroke eng.: η ind,HP 38 4. Results: PMEP % WOT; 4 cylinder engine 39 4. Results: Mechanical Efficiency Chen-Flynn; WOT; 4 cylinder engine 40 4. Results: Compressor Power % WOT; 4 cylinder engine 41 4. Influence of Scavenging Considered Scavenging Models hPerfect Mixing – idealized case of equal distribution of all species in a cylinder hUniFlow – the best case => no mixing of fresh mixture with exhaust gases hBad Mixing (Shortcut Scavenging) – the worst case => fresh mixture can flow immediately into an 42 exhaust port 4. Scavenging model influence WOT; 4-cylinder 43 4. Results: BSFC (3-cylinder) Complete engine map; new FMEP; unlim. IGR: 44 4. Constraints: Peak Pressure Complete engine map; new FMEP; unlim. IGR: 45 4. Constraints: Free O2 (3-cylinder) Complete engine map; new FMEP; unlim. IGR: 46 4. Constraints: IGR % (3-cylinder) Complete engine map; new FMEP; unlim. IGR: 47 4. Constraints: IGR % (3-cylinder) Complete engine map; new FMEP; unlim. IGR: 48 4. Results: Boost Pressure (3-cylinder) Complete engine map; new FMEP; unlim. IGR: 49 4. Results: TDC Temperature Complete engine map; new FMEP; unlim. IGR: 50 5. Conclusions: • 2-stroke engine concept was compared with 4-stroke one applying calibrated simulation tools (GT-Power and modeFRONTIER). • When both engine concepts are compared under the same operating conditions (full load), 4-stroke supercharged engine is clearly superior to 2-stroke one for the case of passenger car small engine => the difference is usually more than 20g/kW/h (full load). 51 5. Conclusions disadvantages of 2-stroke concept: • Higher requirement for boost pressure (due to shorter time of valve being open) => more energy is needed to compress inlet mixture. • No possibility to recover some energy (needed to compress inlet mixture) unlike in the case of supercharged 4-stroke engine. • No turbocharging is applicable due to increase of exhaust backpressure. • The main problem of 2-stroke engine at lower engine loads is internal52 EGR (good for HCCI). 5. Conclusions advantages of 2-stroke concept: • 2-stroke engine has potential for better mechanical efficiency. • 2-stroke engine indicated efficiency is mostly higher even though lower IMEP in comparison to a 4-stroke engine. • Due to requirement for less than 5% of free oxygen in exhaust gas, the influence of 2- stroke engine scavenging features is small.
Details
-
File Typepdf
-
Upload Time-
-
Content LanguagesEnglish
-
Upload UserAnonymous/Not logged-in
-
File Pages54 Page
-
File Size-