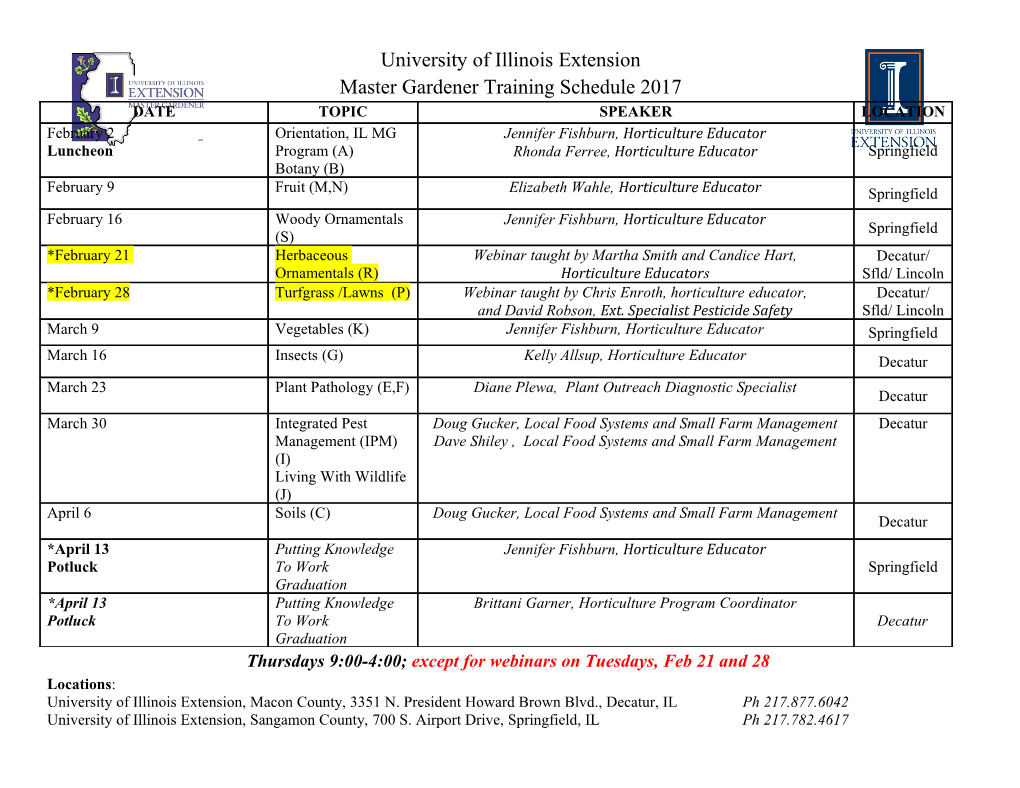
Tobias Großmann Whispering-Gallery-Mode Lasing in Polymeric Microcavities Whispering-Gallery-Mode Lasing in Polymeric Microcavities Zur Erlangung des akademischen Grades eines DOKTORS DER NATURWISSENSCHAFTEN von der Fakult¨at fur¨ Physik des Karlsruher Instituts fur¨ Technologie (KIT) genehmigte DISSERTATION von Dipl.-Phys. Tobias Großmann aus Chang-Hua/Taiwan Tag der mundlichen¨ Prufung¨ : 23.11.2012 Referent : Prof. Dr. Heinz Kalt Korreferent : Priv.-Doz. Dr.-Ing. Timo Mappes Prufungskommission:¨ Prof. Dr. W. Wulfhekel Prof. Dr. H. Kalt Priv.-Doz. Dr.-Ing. T. Mappes Prof. Dr. A. Mirlin Prof. Dr. W. de Boer Prof. Dr. J. Kuhn¨ Karlsruher Institut fur¨ Technologie (KIT) Institut fur¨ Angewandte Physik Wolfgang-Gaede-Straße 1 76131 Karlsruhe http://www.aph.kit.edu/kalt Tobias Großmann [email protected] This work was performed in close collaboration between the Institut fur¨ Angewandte Physik and the Institut fur¨ Mikrostrukturtechnik at the Karlsruher Institut fur¨ Tech- nologie (KIT). Financial support was provided by the Deutsche Telekom Stiftung, the Karlsruhe School of Optics and Photonics (KSOP), the Karlsruhe House of Young Scientists (KHYS), and the DFG Research Center for Functional Nanostruc- tures (CFN), by a grant from the Ministry of Science, Research, and the Arts of Baden-Wurttemberg¨ (Grant No. Az:7713.14-300) and by the German Federal Min- istry for Education and Research BMBF (Grant No. FKZ 13N8168A). Contents 1 Introduction1 2 Microgoblets as whispering galleries 5 2.1 Whispering-gallery modes in optical microcavities ........... 5 2.1.1 Classification of WGMs ..................... 8 2.1.2 Optical properties of microcavities ............... 8 2.2 Passive high-Q microgoblet resonators ................. 14 2.2.1 Lithographic fabrication of microgoblets ............ 15 2.2.2 Resonator-waveguide coupling .................. 17 2.2.3 Experimental setup for tapered optical fiber coupling . 19 2.2.4 Q-factor and modal Structure of WGMs in microgoblets . 21 2.2.5 Finite element simulations of WGMs in microgoblets . 24 2.3 Summary and conclusions ........................ 27 3 Low-threshold microgoblet dye lasers 29 3.1 Dye laser basics .............................. 29 3.1.1 Chemical structure of organic dyes ............... 30 3.1.2 Free-electron model ........................ 31 3.1.3 Optical transitions in dye molecules . 32 3.1.4 Dyes as gain medium ....................... 34 3.2 Dye-doped microgoblet lasers ...................... 38 3.2.1 Rhodamine-doped PMMA .................... 39 3.2.2 Rhodamine-doped microgoblets ................. 40 3.2.3 Pyrromethene-doped microgoblets ................ 46 3.3 Microgoblet lasers for label-free biosensing . 50 3.3.1 Optical biosensors ......................... 51 3.3.2 High-Q WGMs for label-free molecule detection . 52 3.3.3 Microgoblet lasers in aqueous environments .......... 57 3.3.4 Label-free detection of proteins . 61 3.4 Summary and conclusions ........................ 64 I 4 Microgoblet lasers with extrinsic gain layer 65 4.1 Dip-pen nanolithography on microgoblets ................ 66 4.1.1 DPN for surface functionalization of microgoblets . 66 4.1.2 DPN for active microgoblets ................... 69 4.1.3 Integration of gain and biofunctionality in phospholipid-coated microgoblets ............................ 70 4.1.4 Summary and conclusions .................... 71 4.2 Low-threshold lasing in organic semiconductor microgoblets . 72 4.2.1 Guest-host organic semiconductors ............... 73 4.2.2 Simulation of WGMs with organic gain layer .......... 74 4.2.3 Lasing properties of organic semiconductor microgoblets . 76 4.2.4 Summary and conclusions .................... 79 5 Photonic molecule lasers for enhanced light-matter interaction 81 5.1 Whispering-gallery modes in photonic molecules . 82 5.1.1 Classification of supermodes in PMs .............. 83 5.1.2 Supermodes in coupled polymeric microdisks . 85 5.2 Fabrication of polymeric photonic molecules .............. 86 5.3 Spectroscopy of supermode lasing .................... 89 5.4 Vernier effect in photonic molecule lasers . 91 5.5 Photonic molecules for sensing applications ............... 93 5.5.1 Summary and conclusions .................... 95 6 Summary 97 A Nanoporous liquid-core lasers 101 Publications 105 References 109 Acknowledgements 125 II Chapter 1 Introduction Nearly a century after Einstein's theoretical description of the process of stimu- lated emission in 1916 [1], which formed the basis for first experimental realizations of lasers by Townes and Maiman in the 1960s [2, 3], the development of novel de- vices and applications based on lasers are nowadays still a hot topic in science and technology [4, 5]. One of the first type of cavities used to generate coherent laser light through stimulated emission was a so-called whispering-gallery-mode resona- tor, where the light is confined via repeated total internal reflection at the boundary of the dielectric structure, forming a whispering-gallery mode (WGM). Shortly after the first experimental realization of a laser by Maiman, Garret et al. reported in 1961 whispering-gallery-mode lasing from polished spheres made of doped calcium fluoride with diameters in the order of several millimeters [6]. Over the last years, advances in micro- and nano-fabrication have enabled continuous down-scaling of these whispering-gallery-mode lasers. Devices with low-threshold or even thresholdless lasers have been proposed and demonstrated as efficient sources of radiation that operate at room temperature and occupy a small volume on a chip [5, 7,8]. Besides the investigation of lasing, the ability to achieve an ultra-high resonator quality in combination with a small mode volume has made WGM microcavities highly promising for a broad range of applications and scientific research, such as non-linear optics [9{11], cavity quantum electrodynamics (c-QED) [12{14], optical telecommunications [15,16] and sensing [4,17,18]. One of the driving technological breakthroughs leading to the broad utilization of WGM resonators was the development of surface-tension induced cavity geometries, which were first demonstrated for the case of microspheres made from silicon dioxide (SiO2)[19,20] and later transferred to on-chip resonators in the form of microtoroids [21, 22]. Microcavities fabricated with a surface-tension induced reflow step can 1 Chapter 1. Introduction possess an atomic-scale surface finish [21], which leads to extremely long photon lifetimes in the cavities. Unfortunately, the reflow process of SiO2 has to occur with a high-power carbon dioxide (CO2) laser. This process has two major disadvantages. ◦ Firstly, the CO2 laser produces temperatures above 1000 C on the silicon chip, which hinders the additional integration of further components onto the same wafer. Secondly, the reflow process using laser radiation is a serial fabrication technique, so that microtoroids can hardly be produced on a large scale. These limitations can be overcome by the employment of thermoplastic polymers with a low glass-transition temperature (slightly above 100 ◦C), such as poly(methyl methacrylate) (PMMA), as cavity material instead of SiO2. This allows for thermal reflow at temperatures which are around one order of magnitude lower compared to temperatures needed for reflow of SiO2 and therefore enables large-scale fabrication of polymeric devices [23]. Besides this, polymers have the advantage of being low- cost materials [24] which can easily be tailored for different applications, e.g., by integration of additional functional chemical components or gain media [25]. Low material costs and large-scale fabrication in combination with a surface-tension induced cavity geometry make the utilization of polymeric whispering-gallery-mode resonators highly attractive for biosensing applications where the development of disposable sensor chips is of major interest [26]. These biosensors for the label-free detection of molecules are promising for the point-of-care diagnosis, where the en- tire analysis system is ideally integrated in a compact hand-held device. This high integration level is one of the primary properties for future label-free biosensors [27]. So far, experiments with passive WGM resonators with high quality factors demon- strated a sensitivity down to the single molecule level [17]. Despite this promising prospect of an extremely high sensitivity, the technology used for the excitation of WGMs in passive microcavities via tapered fibers is a major drawback for the de- velopment of commercial products based on passive, on-chip devices. Tapered fibers can degrade within hours and require a positioning precision on the nanometer scale. One solution to overcome the aforementioned technological difficulties of operating passive high-Q microresonators is the use of active microcavities instead of passive ones. In addition to the possibility of using the robust free-space excitation and detection of lasing modes, active microcavities in principle allow for an even higher detection sensitivity compared to their passive counterparts. This originates from the narrower linewidths achievable with active cavities due to the compensation of propagation losses in the presence of gain [28, 29]. Therefore, in combination with the cost advantage of polymers, efficient on-chip polymeric WGM lasers are one of the most attractive approaches for the realization of compact systems for label-free molecule detection. 2 To achieve a high detection sensitivity utilizing photonic microstructures, two dif- ferent strategies
Details
-
File Typepdf
-
Upload Time-
-
Content LanguagesEnglish
-
Upload UserAnonymous/Not logged-in
-
File Pages131 Page
-
File Size-