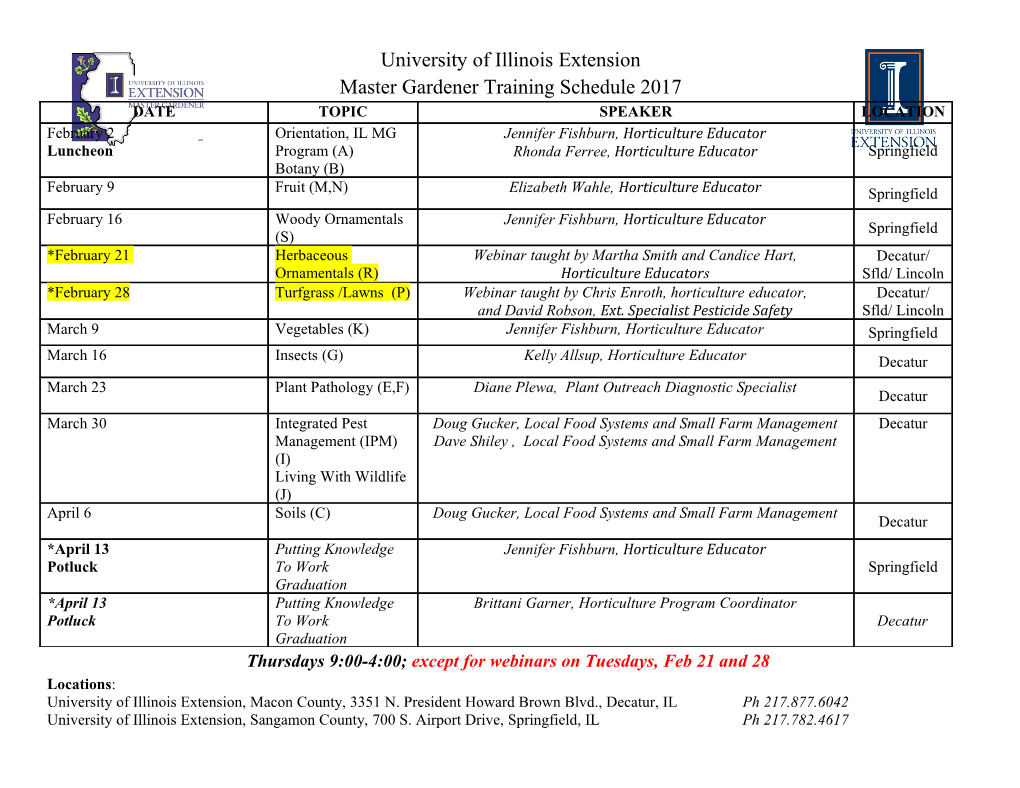
Ben Heidenreich Lecture notes, 3/31/2010 The Five Superstring Theories So far, we have found two superstring theories in ten dimensions: type IIA and type IIB string theory, which are related by T-duality. These nicely correspond to the two = 2 supergravity theories in 10D, of N the same names. Are there more superstring theories? If so they should have corresponding supersymmetric low energy effective actions. Assuming 10D Lorentz invariance, what is left? Well, all = 1 theories. N N = 1 Supergravity For = 1 10, there are 16 supercharges, and two massless multiplets. The supergravity multiplet contains N µ the gravition gµν, dilaton Φ, two-form Bµν, gravitino Ψ α and dilatino λ α. In SO(8) notation, the bosonic sector is [0] + [2] + (2) = 1 + 28 + 35 and the fermionic sector is 56 + 8 ′, for a total of 64 states in each ′ sector. The gauge multiplet contains a gluon Aµ and gluino χα , i.e. 8 v + 8 (an ultrashort multiplet). Thus, to specify the spectrum of the theory, we need only specify the “gluon” gauge group. This also suf- fices to determine the effective action, whose bosonic portion is: 2 − Φ/2 1 1 1 − κ e S = d10 x √ g R ( Φ) 2 e Φ H 2 Tr F2 2 κ2 − − 2 ∇ − 2 | | − 2 g2 Z " # where F = d A iA A − ∧ κ2 H = d B Ω − g2 3 2 i Ω = Tr A d A A A A 3 ∧ − 3 ∧ ∧ Note that the “Yang-Mills” coupling, g, is dimensionful, but the combination g4/κ3 is dimensionless. How- ever, this combination can be shifted by shifting the dilaton, so in fact the action contains one dimen- sionful scale, and no adjustable parameters. Classically, we can choose any gauge group. However, most gauge groups (including pure = 1 super- N gravity) will be anomalous. It turns out that the only anomaly free gauge groups are the following: Spin( 32)/Z E E 2 8 × 8 E U(1) 248 U(1) 496 8 × Here Spin( 32)/Z 2 is essentially SO( 32) (they have the same Lie algebra, but different global properties), and E8 is the rank 8, dimension 248 exceptional (simple) Lie group. Note that all of these groups have dimension 496. In addition, the first two both have rank 16, and con- tain SO( 16) SO( 16) as a subgroup. The later two have rank 256 and 496 respectively. It turns out that × there are string theories corresponding to the Spin( 32)/Z and E E cases (in fact, two for Spin( 32)/ 2 8 × 8 Z2 ), though not for the latter two groups. How can we construct these string theories? So far we have considered only closed, oriented string theo- ries. A straightforward generalization is to consider closed+open and/or unoriented theories (with the same worldsheet action as before). 1 Ben Heidenreich Lecture notes, 3/31/2010 Unoriented strings and orientifolds What are unoriented strings? Define a world-sheet parity operator Ω by: Ω : σ σ → − This exchanges left and right movers. Thus, for instance, the NS-R and R-NS sectors are mapped into each other. In type IIB this is a global symmetry, since these sectors are identical. In type IIA, however, Ω must be combined with spatial parity in order to obtain a global symmetry, since these sectors have opposite parities. To obtain unoriented strings, we gauge Ω (or Ω + parity). In the type IIB case, it is easy to see that in the NS-NS sector, this removes Bµν and leaves gµν and Φ. The NS-R and R-NS sectors are identified, leaving the same fermion content as the = 1 SUGRA multiplet. Thus, we must have a total of 64 N bosonic states. The only way to achieve this is if Cµν is projected in an C0 and Cµνρσ are projected out (one can verify this in a more fancy manner...) ′ Now consider the IIA case. We require a Z2 involution Σ: x x which reverses spatial parity. For con- creteness, consider Σ : x9 x9. Thus, away from the fixed→ plane, have ordinary type IIA. At the fixed → − plane, we find that Bµν and gµ 9 are projected out, whereas g99, gµν, Bµ 9, and Φ are projected in. The NS-R and R-NS sectors are identified as before, so we still need a total of 64 bosonic states. We have at present [0] + [0] + [1] + (2) = 1 + 1 + 7 + 27 in reps of SO(7) . The rest of the multiplet is logically filled out by [1] + [3] = 7 + 21 , which can be explained if Cµ and Cµν 9 are projected in, whereas C9 and Cµνρ are projected out. In general this construction (gauging a combination of a Z 2 involution and world-sheet parity) is called an orientifold . For type IIB, the involution must preserve spatial orientation, and for IIA it must reverse spa- tial orientation. The simplest orientifold is the trivial involution for IIB (the only one which results in a Lorentz invariant theory), but in general the involution can be complicated. The fixed planes of the invo- lution are called orientifold fixed planes, or Op planes (e.g. O9 planes for the spacetime filling case in IIB). However, the orientifold of IIB is anomalous... will need to add a gauge multiplet... Open Strings What about open strings? Adding open strings to the spectrum is equivalent to adding any number/con- figuration of Dp branes. For instance, the simplest case is to add a single D9 brane (Neumann BCs). What if we add multiple D9 branes? This leads to “Chan-Paton” factors, i.e. labels carried at each end of an open string which indicate which of the coincident D9 branes the string ends on. If we compute e.g. scattering amplitudes, one finds that the open string vector Aµ, which carries two Chan-Paton labels (one for each end) now lives in a gauge group U( N) (where N is the number of D9 branes). Why U( N) ? The massless level states are: µ µ,ij ψ− p,i,j A 1/2 | i i, j X Choose as basis N2 Hermitean N N matrices: × a a µ,ij = A λ A µ ij a X a a b ab The λ ij are the generators of the U( N) Lie algebra, where Tr( λ λ ) = δ ... so we have gauge group U( N) . 2 Ben Heidenreich Lecture notes, 3/31/2010 What about unoriented open strings? Ω must exchange i and j. Turns out there is a sign reversal too: µ µ Ω ψ− p,i,j = ψ− p,j,i 1/2 | i − 1/2 | i In this case, we must choose the λ a to be antisymmetric, in which case we obtain the gauge group SO( N) . More generally, can combine Ω with a U( N) rotation γij on the Chan-Paton factors: µ µ − 1 Ω ψ− p,i,j = ǫγil ψ− p,k,l γ 1/2 | i 1/2 | i kj where ǫ is a phase. Since Ω must square to the identity, 2 µ µ − 1 − 1 Ω ψ− p,i,j = ǫγil ǫγkn ψ− p,m,n γ γ 1/2 | i 1/2 | i ml kj 2 T − 1 µ T − 1 = ǫ γ ( γ ) ψ− p,m,n γ γ im 1/2 | i nj Thus, γ must be either symmetric or antisymmetric, and ǫ2 = 1 so ǫ is a sign. The generators λ, will have to satisfy: λ = ǫγλ T γ− 1 Commutator of two λ’s: 2 T T − 1 [ λ 1 , λ2 ] = ǫ γ λ 1 , λ 2 γ = γ [ λ , λ ] Tγ− 1 − 1 2 Thus, to obtain a Lie algebra (closed under commutation) must choose ǫ = 1 . What does the corre- sponding Lie group look like (require λ’s to be Hermitean)? Well, we have λγ =− γλ T. Thus, − T eiλ γ eiλ = eiλ e− iλ γ = γ so the Lie group preserves the symmetric (antisymmetric) nondegenerate matrix γ. This leads to the Lie groups SO( N) and USp( N) respectively (both simple Lie groupds, and subgroups of U( N) ). Call the O plane which produces SO( N) an O9 − plane, and that for USp( N) an O9+ plane. Note that for the USp( N) case, N will have to be even. Type I superstring theory Now consider adding open strings to the spectrum on either type II string theory. We will need to do a GSO projection in the open string sector. There are two choices: NS + , R + : 8 v + 8 ′ NS + , R : 8 v + 8 − These are both multiplets of = 1 SUGRA but not of = 2 SUGRA, so we must combine the open and unoriented theories. Thus, takeN the IIB orientifold and addN an open string sector (must be the NS + , R + GSO projection for consistency). We can add any number of D-branes/Chan-Paton factors, and use either an O9+ or an O9 − , obtaining either a symplectic or orthogonal gauge group. However, the only nonanomalous case is 32 D9’s + O9− , with gauge group SO( 32) . This is Type I superstring theory, and gives the low energy effective theory = 1 , Spin( 32)/Z SUGRA. N 2 3 Ben Heidenreich Lecture notes, 3/31/2010 D branes The existence of the type I theory tells us a lot about D-branes in superstring theories via T-duality. In particular, consider the T-dual theory to type I compactified on a circle (known as type I ′). Recall that T- duality replaces: 9 9 9 X = XL + XR with X˜ 9 = X9 X9 L − R The world-sheet parity operator Ω exchanges left and right movers, so: Ω : XL XR ↔ Thus Ω : X˜ 9 X˜ 9 → − Thus, on the T-dual side, we find an orientifold of type IIA with a nontrivial Z involution X˜ 9 X˜ 9.
Details
-
File Typepdf
-
Upload Time-
-
Content LanguagesEnglish
-
Upload UserAnonymous/Not logged-in
-
File Pages14 Page
-
File Size-