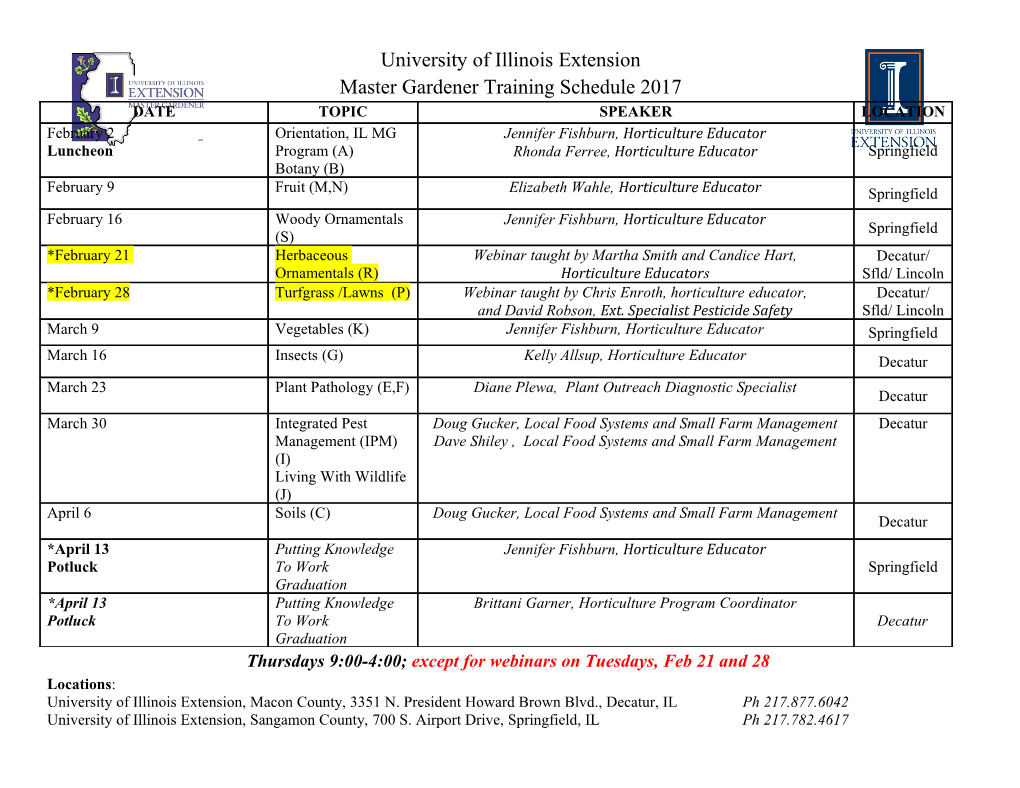
Landscape and Urban Planning 177 (2018) 227–240 Contents lists available at ScienceDirect Landscape and Urban Planning journal homepage: www.elsevier.com/locate/landurbplan Research Paper Where the people are: Current trends and future potential targeted T investments in urban trees for PM10 and temperature mitigation in 27 U.S. Cities ⁎ Timm Kroegera, , Robert I. McDonaldb, Timothy Bouchera, Ping Zhangc, Longzhu Wangd a Office of the Chief Scientist, The Nature Conservancy, 4245 North Fairfax Drive, Suite 100, Arlington, VA 22203, USA b Global Cities Program, The Nature Conservancy, 4245 North Fairfax Drive Suite 100, Arlington, VA 22203, USA c NASA Goddard Space Flight Center, Greenbelt, MD 20771, USA d China Regional Office, The Nature Conservancy, Beijing 100600, China ARTICLE INFO ABSTRACT Keywords: Urban trees reduce respirable particulate matter (PM10) concentrations and maximum daytime summer tem- Urban forest peratures. While most cities are losing tree cover, some are considering ambitious planting efforts. Maximizing Particulate matter PM10 and heat mitigation for people from such efforts requires cost-effective targeting. We adapt published Urban heat methods to estimate the impact of a decade (2004–2014) of tree cover change on city-level PM10 and heat Return on investment mitigation in 27 U.S. cities and present a new methodology for estimating local-level PM and heat mitigation Targeting 10 by street trees and tree patches. We map potential tree planting sites in the 27 cities and use our local-level PM Ecosystem services 10 and heat mitigation methods to assess the population-weighted return on investment (ROI) of each site for PM10 and heat abatement for nearby populations. Twenty-three of the 27 cities lost canopy cover during 2004–2014, reducing estimated city-level PM10 removal by 6% (168 tons) and increasing city-level average maximum daily summer temperature by 0.1 °C on average across cities. We find large potential for urban reforestation to in- crease PM10 and heat abatement. Intra-city variation in planting site ROI – driven primarily by differences in population density around planting sites – exceeds four orders of magnitude, indicating large scope for targeting to increase PM10 and heat abatement from reforestation. Reforesting each city’s top 20% ROI sites could lower 3 average annual PM10 concentrations by > 2 μg/m for 3.4–11.4 million people and average maximum daily summer temperatures by > 2 °C for 1.7–12.7 million – effects large enough to provide meaningful health benefits – at a combined annual cost of $102 million. 1. Introduction mitigation per dollar spent. Yet, despite the increasing recognition of the pollution and heat mitigating effects of urban trees, many cities in Large-scale increases in urban vegetation cover have been suggested the U.S. are experiencing declines in urban tree canopy (Nowak & as an important strategy for mitigating urban summer temperature Greenfield, 2012), potentially impacting PM concentrations and air extremes (Akbari, Pomerantz, & Taha, 2001; Taha, Konopacki, & temperature. Gabersek, 1999) and reducing premature heat-related mortality (Stone In this paper, we quantify how current trends in urban tree canopy et al., 2014). Strategic deployment of urban vegetation additions also is cover have impacted urban air quality and summer temperatures, and considered highly effective for reducing local airborne particulate assess the potential for targeted investments in tree cover gains to im- matter (PM) concentrations (Maher, Ahmed, Davison, Karloukovski, & prove these two parameters. In this section, we present basic back- Clarke, 2013; Pugh, Mackenzie, Whyatt, & Hewitt, 2012) and enhan- ground information on what is known about urban tree canopy miti- cing the PM-related mortality and morbidity reductions urban tree gation of PM and temperature, and then present our specific research cover provides (Nowak, Hirabayashi, Bodine, & Hoehn, 2013). Indeed, goals. urban planners increasingly consider vegetation management for air pollution and heat mitigation (Andersson-Sköld et al., 2015), though rarely in a systematic manner that maximizes population-weighted ⁎ Corresponding author. E-mail addresses: [email protected] (T. Kroeger), [email protected] (R.I. McDonald), [email protected] (T. Boucher), [email protected] (P. Zhang), [email protected] (L. Wang). https://doi.org/10.1016/j.landurbplan.2018.05.014 Received 28 November 2016; Received in revised form 23 October 2017; Accepted 15 May 2018 Available online 26 May 2018 0169-2046/ © 2018 The Author(s). Published by Elsevier B.V. This is an open access article under the CC BY-NC-ND license (http://creativecommons.org/licenses/BY-NC-ND/4.0/). T. Kroeger et al. Landscape and Urban Planning 177 (2018) 227–240 1.1. Urban tree effects on particulate matter greenspace-level PM concentration reduction studies (e.g., Cavanagh, Zawar-Rezab, & Wilson, 2009; Cowherd, Muleski, & Gebhardt, 2006; Airborne particulate matter is widely considered the most pressing Maher et al., 2013; Tiwary, Reff, & Colls, 2008; Zhu et al., 2011), and urban air quality problem globally and is a key factor in climate change by land use regression and other spatial-statistical analyses examining (Fuzzi et al., 2015). In U.S. urban outdoor environments, the main the relationship between PM concentrations and urban forests or other contributors to ambient concentrations of respirable particles (< 10 μm green areas (Eeftens et al., 2012; Irga, Burchett, & Torpy, 2015; Ross, in aerodynamic diameter, or PM10) generally are agriculture, traffic Jerrett, Ito, Tempalski, & Thurston, 2007; Wu, Xie, Li, & Li, 2015; Xu (fuel combustion; road, tire and break wear), household fuel burning et al., 2016). and powerplant and industrial emissions (Bauer, Tsigaridis, & Miller, Some modeling studies have shown that dense canopies in high- 2016; Hopke et al., 2006; Karagulian et al., 2015; Sowlat, emission street canyons under certain conditions can increase local PM Hasheminassab, & Sioutas, 2016), from local, regional and long-range concentrations if their aerodynamic effect (reduced ventilation and thus sources (Karnae & Kuruvilla, 2011; Kundu & Stone, 2014; Levy & pollutant dispersion) exceeds their pollutant removal effect (Janhäll, Hanna, 2011). In the western U.S., wildfires can be a seasonally im- 2015; Pugh et al., 2012; Vos, Maiheu, Vankerkom, & Janssen, 2013). portant source as well (Spracklen et al., 2009). Due to the high particle However, which of the two effects prevails depends on the microscale infiltration and indoor penetration factors of PM10 (Chen & Zhao, (leaf-level) deposition velocity used in the modeling (Santiago, Martilli, 2011), outdoor PM10 also is a major contributor to indoor PM10 con- & Martin, 2017), which in some studies may be set unrealistically low centrations (Almeida et al., 2011; Rovelli et al., 2014). (Maher et al., 2013; Santiago et al., 2017). Empirical evidence shows While coarse particles between 2.5 and 10 μm in aerodynamic dia- that such pollution “trapping” by dense streetside canopies may (Chen meter (PM10-2.5) are suspected to have similar health effects, evidence et al., 2015) or may not (Hofman, Stokkaer, Snauwaert, & Samson, of increased cardiovascular and respiratory morbidity and mortality 2013) occur at heights where human exposure occurs. Roadside treed from short-term and long-term exposure is strongest for fine particles areas experiencing high pedestrian use therefore should be managed for (PM2.5)(Correia et al., 2013; Hoek et al., 2013; Thurston et al., 2016; less dense canopy crowns, which facilitate pollutant dispersion while U.S. EPA, 2009) with specific pathways for cardiovascular effects re- still achieving high PM removal efficiency (Maher et al., 2013). cently demonstrated (Kaufman et al., 2016). In fact, PM2.5 is considered the dominant contributor to the health burden from outdoor air pol- 1.2. Urban tree effects on summer maximum daily air temperature lution both in the U.S. (Fann et al., 2012) and globally, being re- sponsible for an estimated 3.2 million (M) premature deaths per year Near-surface temperatures in cities generally significantly exceed worldwide (Lim & et al., 2012). PM2.5 has been linked to neurodegen- those in surrounding rural areas (Arnfield, 2003; Bounoua et al., 2015; erative disease and Alzheimer’s disease (Jung, Lin, & Hwang, 2015; Imhoff, Zhang, Wolfe, & Bounoua, 2010). This “urban heat island” Maher et al., 2016), and PM10 concentrations have a significant nega- (UHI) phenomenon is caused primarily by two factors: the replacement tive effect on worker productivity (Chang, Graff Zivin, Gross, & Neidell, of vegetation with impervious surfaces that have higher heat absorption 2016) and cognitive performance and development in children, re- capacity and produce no evaporative cooling; and the release of waste sulting in lower academic test scores and reduced lifetime earnings heat from anthropogenic processes like motor vehicle operation and air potential (Clark-Reyna, Grineski, & Collins, 2016; Dadvand et al., 2015; conditioning (Arnfield, 2003). The intensity of the UHI effect is sig- Freire et al., 2010; Lavy, Ebenstein, & Roth, 2014; Suglia, Gryparis, nificantly positively related to both impervious surface fraction and city Wright, Schwartz, & Wright, 2008). size (Bounoua et al., 2015; Imhoff et al., 2010). Over the last 30 years, regulatory controls have achieved large re- While the UHI effect is observed in both summer and winter ductions in urban PM concentrations (Darlington, Kahlbaum, Heuss, & (Bounoua
Details
-
File Typepdf
-
Upload Time-
-
Content LanguagesEnglish
-
Upload UserAnonymous/Not logged-in
-
File Pages14 Page
-
File Size-