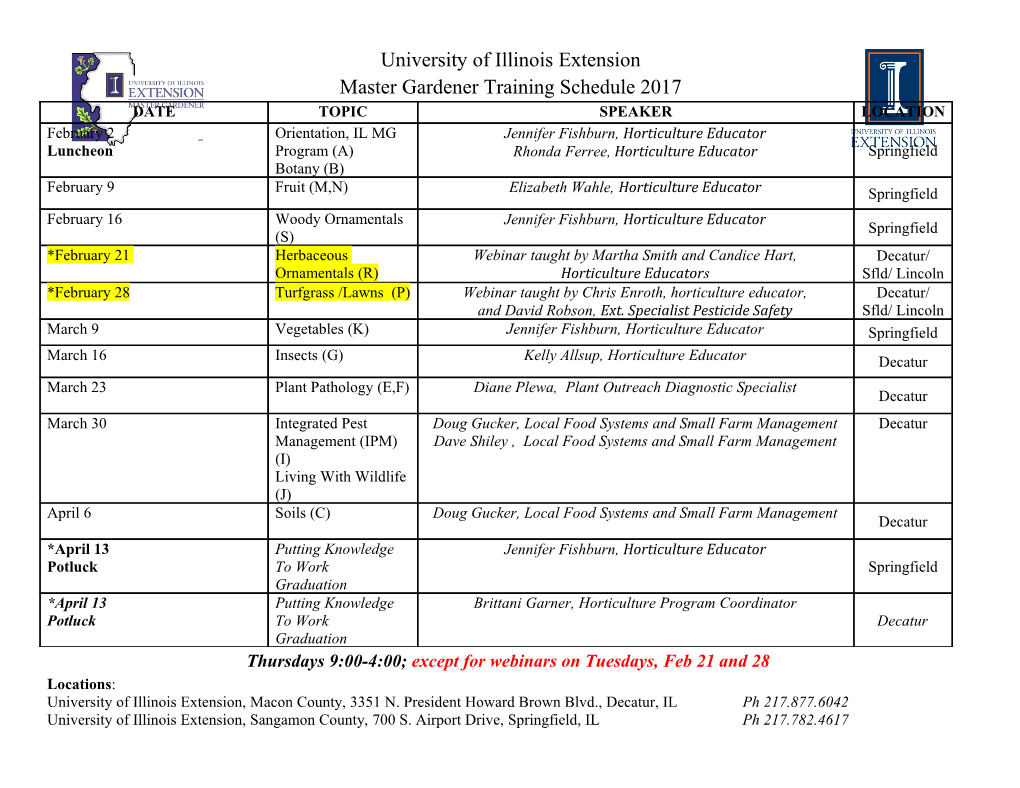
Rapid chemotactic response enables marine bacteria to exploit ephemeral microscale nutrient patches Roman Stocker*†, Justin R. Seymour*, Azadeh Samadani‡, Dana E. Hunt*, and Martin F. Polz* *Department of Civil and Environmental Engineering, Massachusetts Institute of Technology, 77 Massachusetts Avenue, Cambridge, MA 02139; and ‡Department of Physics, Brandeis University, 415 South Street, Waltham, MA 02454 Edited by David M. Karl, University of Hawaii, Honolulu, HI, and approved January 22, 2008 (received for review October 14, 2007) Because ocean water is typically resource-poor, bacteria may gain of bacterial clusters around sporadic protist lysis events (2) and significant growth advantages if they can exploit the ephemeral nutrient-loaded beads (11). The potential quantitative impor- nutrient patches originating from numerous, small sources. Al- tance of these microscale interactions has emerged from theo- though this interaction has been proposed to enhance biogeo- retical models (5–7), yet the study of environmentally realistic chemical transformation rates in the ocean, it remains questionable patch-evolution scenarios has been hampered by technical lim- whether bacteria are able to efficiently use patches before physical itations in the laboratory and by the small scale and intermit- mechanisms dissipate them. Here we show that the rapid chemo- tency of patch dynamics in situ (12). tactic response of the marine bacterium Pseudoalteromonas halo- Here, we ask to what extent marine bacteria are capable of planktis substantially enhances its ability to exploit nutrient exploiting ephemeral nutrient patches generated at environmen- patches before they dissipate. We investigated two types of tally realistic spatiotemporal scales. We use microfluidic devices patches important in the ocean: nutrient pulses and nutrient to generate two types of patches that are expected to occur plumes, generated for example from lysed algae and sinking widely in the ocean (9): a purely diffusive pulse and a plume organic particles, respectively. We used microfluidic devices to governed by diffusion and advection. Microfluidic techniques create patches with environmentally realistic dimensions and dy- have previously been used to gain insight into different aspects namics. The accumulation of P. haloplanktis in response to a of microbial ecology, including chemotaxis (13) and population nutrient pulse led to formation of bacterial hot spots within tens behavior within complex landscapes (14, 15). Here, we have of seconds, resulting in a 10-fold higher nutrient exposure for the fabricated microchannels that create patches with spatial and fastest 20% of the population compared with nonmotile cells. temporal scales consistent with those expected in the ocean. By Moreover, the chemotactic response of P. haloplanktis was >10 simultaneously measuring the spatiotemporal distribution of times faster than the classic chemotaxis model Escherichia coli, nutrients and cells, we quantified the nutrient exposure experi- leading to twice the nutrient exposure. We demonstrate that such enced by chemotactic marine bacteria. rapid response allows P. haloplanktis to colonize nutrient plumes for realistic particle sinking speeds, with up to a 4-fold nutrient Results exposure compared with nonmotile cells. These results suggest Diffusing Nutrient Pulse. We examined the chemotactic response ␥ that chemotactic swimming strategies of marine bacteria in patchy to a diffusing nutrient patch of the marine -proteobacterium nutrient seascapes exert strong influence on carbon turnover rates Pseudoalteromonas haloplanktis, which has previously served as by triggering the formation of microscale hot spots of bacterial a model of chemotaxis (11). We simulated a nutrient pulse by productivity. using a microinjector (Fig. 1a), which generated a one- dimensional (1D), 300-m wide band of nutrients (Fig. 1b), chemotaxis ͉ marine snow ͉ microfluidics ͉ patchiness mimicking the sudden release of organic matter associated with viral lysis or sloppy predation of phytoplankton cells (2). The chemoattractant consisted of culture filtrates from the marine arine bacteria inhabit a complex environment where nu- phytoplankton Dunaliella tertiolecta. Although patch composi- Mtrients are typically concentrated in small (micrometers to tion in the ocean is poorly characterized, phytoplankton-derived centimeters), often ephemeral, patches (1–4), which can contain DOM was chosen because it is likely a significant nutrient biologically labile organic compounds at concentrations two to component of many patches (4, 5, 16, 17). Algal-derived dis- three orders of magnitude above ambient seawater (2, 5, 6). solved organic carbon (DOC) concentration in the culture Sources of transient patches include phytoplankton photosyn- filtrates (567 M) was on the high end of measured bulk DOC thetic products (4, 7), zooplankton excretion (8), cell lysate (2), concentrations during phytoplankton blooms (e.g., ref. 18), and organic matter leaking from particles (6). Although indi- representative of conditions in a patch. We use the response to SCIENCES vidual patches of dissolved organic matter (DOM) are small, model chemoattractants as indicative of the response to nutri- ENVIRONMENTAL they are abundant; hence the efficiency of marine bacteria in ents and hereafter employ the words ‘‘nutrient’’ and ‘‘chemoat- using nutrient patches exerts a fundamental control on biogeo- tractant’’ interchangeably. In the microfluidic channel, bacteria chemical transformation rates in the ocean (1, 9). However, it has were advected along both sides of the nutrient band. The never been quantitatively evaluated whether the chemotactic experiment was initiated by turning off the flow (t ϭ 0), causing response of marine bacteria is rapid enough to enable significant fluid motion to stop instantaneously and nutrients to diffuse gain from nutrient patches in the face of their rapid physical laterally. Thereafter, we recorded the across-channel (x direc- dissipation. Motile bacteria can secure a significant advantage from a transient nutrient source only if the biological time scale (BIO) Author contributions: R.S., J.R.S., and M.F.P. designed research; R.S., J.R.S., and A.S. per- formed research; R.S. analyzed data; and R.S., J.R.S., D.E.H., and M.F.P. wrote the paper. of their response is shorter than the time scale (PHYS) over which physical processes homogenize the patch, i.e., if BIO Ͻ PHYS. The authors declare no conflict of interest. For stationary nutrient pulses generated by a lysing cell or a This article is a PNAS Direct Submission. sloppy feeding event, PHYS is set by diffusion, whereas for †To whom correspondence should be addressed. E-mail: [email protected]. moving sources such as a sinking particle, PHYS is further This article contains supporting information online at www.pnas.org/cgi/content/full/ influenced by advection (10). Attraction to microscale nutrient 0709765105/DC1. patches in the environment has been inferred from observations © 2008 by The National Academy of Sciences of the USA www.pnas.org͞cgi͞doi͞10.1073͞pnas.0709765105 PNAS ͉ March 18, 2008 ͉ vol. 105 ͉ no. 11 ͉ 4209–4214 Downloaded by guest on September 29, 2021 Fig. 1. Microchannels used to probe the chemotactic response of bacteria to nutrient patches and plumes. (a) Microchannel for diffusing nutrient pulse experiments. The two inlets (‘‘in’’) are for bacteria (yellow) and chemoattrac- tant (white). (b) Microinjector tip, corresponding to the red dashed box in a. The 300-m-wide injected nutrient band was visualized by using 100 M Fig. 2. Response to a nutrient pulse. (a) Trajectories of P. haloplanktis, 2 min fluorescein. (c) Cylindrical particle (R ϭ 250 m) used in the nutrient plume after release of a 300-m-wide nutrient pulse (black bar). Each white path is experiments. an individual trajectory. (b) Time evolution of the hot spot index H for P. haloplanktis (blue, with chemoattractant; light blue, control run) and E. coli (red, with chemoattractant; light red, control run). Initially H Ͻ 1 because the tion) distribution of both nutrients C(x) and bacteria B(x) every nutrient band is devoid of bacteria. (c) Mean chemotactic advantage index 13 s for at least 13 min. The nutrient distribution was visualized ACHE over nonmotile cells. The gray background and associated color bar by using fluorescein, which has a diffusivity (D ϭ 0.5 ϫ 10Ϫ9 m2 indicate the mean nutrient concentration within the central 300- m region, normalized by its initial value. Color scheme as in b.(d) Chemotactic advantage sϪ1) typical of low molecular weight substances. Measurements ACHE,i of individual bacteria inside the central 300-m region relative to those of C(x) showed that the patch diffused from an original width of outside. (Insets) The fraction of the population inside the central band at four 300 mtoϷ1 mm within 3 min, at which time only 40% of the points in time (the color scheme is as in b). original nutrients remained within the central 300 m. Because the nutrients diffused outwards, P. haloplanktis cells strongly aggregated at the center of the band (Fig. 2a) within tens nutrient patch than E. coli (D ϭ 7.5 min). This partly reflects a Ϫ1 of seconds, forming a hot spot, which persisted for at least 15 difference in mean swimming speed VB (68 ms for P. min. To quantify the magnitude and time scale of the accumu- haloplanktis;31msϪ1 for E. coli). A quick response is critical lation, we define a hot spot index H as the mean concentration because the mean nutrient concentration inside the central of bacteria within the central 300-m region relative to the mean region decays rapidly, as shown by the background gray shading concentration over the entire channel width. We expect two in Fig. 2b; between 0.5 and 5.0 min, it decreased by 55%. components of bacterial motility to determine H: random mo- Motility significantly enhanced the nutrient exposure of a tility (19), which tends to make the bacterial distribution uniform population of P. haloplanktis, measured by integrating the nu- (H ϭ 1), and chemotaxis, which induces aggregation in the patch trient exposure experienced by each cell, or ͐B(x)C(x)dx. The (H Ͼ 1).
Details
-
File Typepdf
-
Upload Time-
-
Content LanguagesEnglish
-
Upload UserAnonymous/Not logged-in
-
File Pages6 Page
-
File Size-