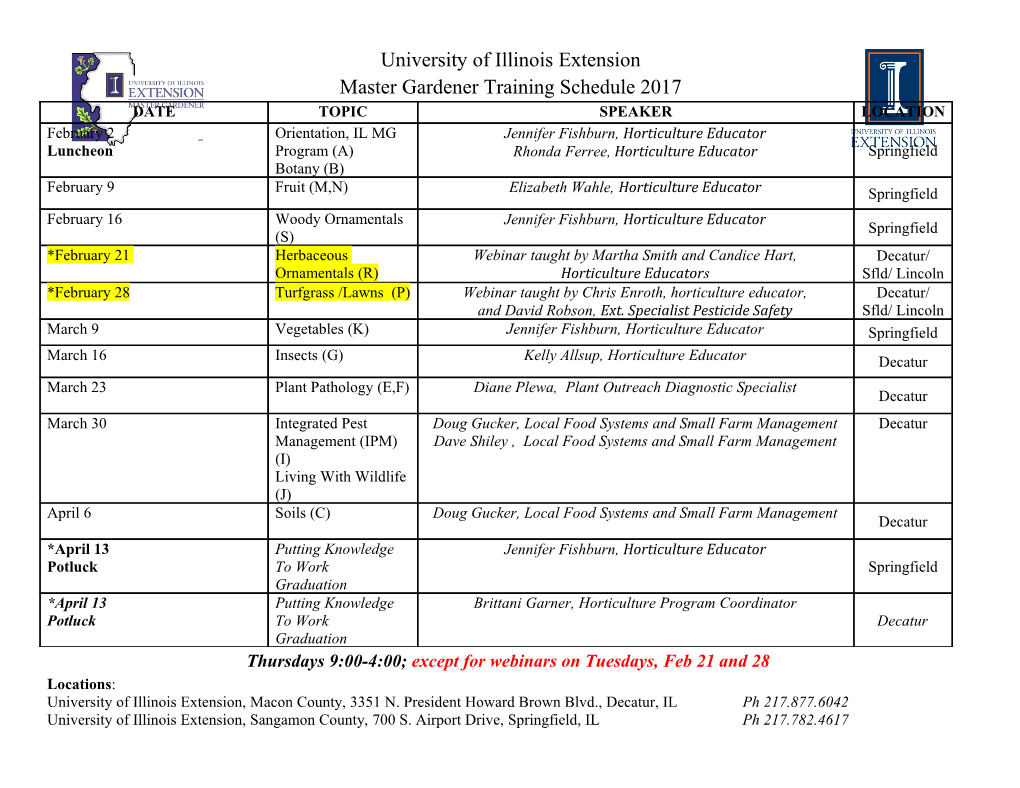
Biotechnol. Prog. 1999, 15, 81−90 81 Modeling of Overflow Metabolism in Batch and Fed-Batch Cultures of Escherichia coli Bo Xu, Mehmedalija Jahic, and Sven-Olof Enfors* Department of Biotechnology, Royal Institute of Technology, S-100 44 Stockholm, Sweden A dynamic model of glucose overflow metabolism in batch and fed-batch cultivations of Escherichia coli W3110 under fully aerobic conditions is presented. Simulation based on the model describes cell growth, respiration, and acetate formation as well as acetate reconsumption during batch cultures, the transition of batch to fed-batch culture, and fed-batch cultures. E. coli excreted acetate only when specific glucose uptake exceeded a critical rate corresponding to a maximum respiration rate. In batch cultures where the glucose uptake was unlimited, the overflow acetate made up to 9.0 ( 1.0% carbon/ carbon of the glucose consumed. The applicability of the model to dynamic situations was tested by challenging the model with glucose and acetate pulses added during the fed-batch part of the cultures. In the presence of a glucose feed, E. coli utilized acetate 3 times faster than in the absence of glucose. The cells showed no significant difference in maximum specific uptake rate of endogenous acetate produced by glucose overflow and exogenous acetate added to the culture, the value being 0.12-0.18 g g-1 h-1 during the entire fed-batch culture period. Acetate inhibited the specific growth rate according to a noncompetitive model, with the inhibition constant (ki) being 9 g of acetate/L. This was due to the reduced rate of glucose uptake rather than the reduced yield of biomass. Introduction nol production (Sonnleitner and Ka¨ppeli, 1986), this process is now known as glucose overflow metabolism. Acetic acid formation by aerobically growing Escheri- High specific growth rate (Majewski and Domach, 1990; chia coli was already documented more than four decades Britten, 1954), high specific glucose uptake rate (Han et ago (Britten, 1954). Since then, there has been an array al., 1992), bottlenecks in the Krebs cycle (Hollywood and of literature reports bringing up the same topic (Farmer Doelle, 1976; Gray et al. 1966; Amarasingham and Davis, and Liao, 1997; Holms, 1996, 1986; Pan et al., 1987; 1965), limited respiratory capacity (Ingledew and Poole, Meyer et al., 1984). In particular, when E. coli was to be 1984), or a combination of any of the above has been considered as a host organism to mass produce important suggested to trigger the acetate overflow metabolism. For human pharmaceutical proteins or other foreign proteins, better understanding and quantitative prediction of the a detailed documentation of acetic acid formation became acetate overflow phenomenon, a number of mathematical important. An example of this is that 19 out of the 23 models (Ko et al., 1993, 1994; El-Mansi et al., 1994; studies on recombinant E. coli reviewed by Lee (1996) Varma and Palsson, 1994; Majewski and Domach, 1990; reported on acetate accumulation during the fed-batch Bajpai, 1987) were reported. Another type of model, using processes. This is also true when the continuous culture visual programming of aerobic acetate formation, was technique was applied (Curless et al., 1989; Brown et al., also proposed (Regan and Gregory, 1995). Most modeling 1985). Acetate produced in a culture has been shown to of E. coli deals with acetate formation only and with inhibit several physiological properties of the culture continuous culture, while the industrial use of E. coli is itself. There is evidence suggesting that acetate acts as mainly based on fed-batch culture, in which acetate is an uncoupler of the proton motive force of E. coli (Axe formed or consumed, depending on the conditions. and Bailey, 1995; Repaske and Adler, 1981). Its proto- nated state diffuses through the lipid membrane of a cell. This paper deals with dynamic modeling of acetate Thereafter it dissociates in the cytoplasm, causing a pH production and subsequent reconsumption during batch decrease (Axe and Bailey, 1995; Luli and Strohl, 1990). and fed-batch cultures of E. coli K-12 strain W3110. It Various genetic and biochemical engineering or combined was found necessary to include the inhibiting effect of strategies have been tried to avoid or reduce acetate acetate on consumption of oxygen and glucose in the formation (Farmer and Liao, 1997; Chou et al., 1994; model. The model is applied directly to results of batch Konstantinov et al., 1990; El-Mansi and Holms, 1989; and fed-batch cultivation experiments. Bajpai, 1987). Today, much knowledge has accumulated concerning Materials and Methods aerobic acetic acid formation in E. coli. Together with its Bacterial Strain. The wild-type E. coli K-12 strain counterpart phenomenon in yeast, i.e., the aerobic etha- W3110 was obtained from the Genetic Stock Center, Yale University (New Haven, CT). The strain was stored at * Corresponding author. Telephone: +0046-8-790 9164. Fax: -80 °C in the below-described mineral medium supple- +0046-8-723 1890. E-mail: [email protected]. mented with 20% glycerol. 10.1021/bp9801087 CCC: $18.00 © 1999 American Chemical Society and American Institute of Chemical Engineers Published on Web 01/05/1999 82 Biotechnol. Prog., 1999, Vol. 15, No. 1 Table 1. Carbon per Carbon Yield on Glucose for rate started to decline. To estimate the biomass, and Biomass (X), Acetate and Carbon Dioxide: Summary of thereby following the proceeding of cultivation, the optical Six Batch Cultures of E. Coli W3110 density of the culture was measured at 500 nm by using - initial carbon yield on carbon aUV vis spectrophotometer (Lot-Oriel, Darmstadt, Ger- expt glucose glucose (mol C/mol C) recoveryd many). a c run concn (g/L) X acetate CO2 (%) Shake flask experiments were performed in 1-L baffled 1 10.01 0.57 0.08 0.41 106 Erlenmeyer flasks containing a medium volume of 100 2 9.19 0.71 0.05 0.32 108 mL. The mineral medium was supplemented with 5 g/L 3 4.03 0.67 0.12 0.28 107 - 4 4.84 0.59 0.10 0.28 97 of glucose plus 0 8 g/L of sodium acetate at pH 7. 5 13.86 0.59 0.08 0.28 95 Cultivations were carried out at 35 °C at a rotating speed 6 7.47 0.72 0.12 0.29 113 of 200 rpm. mean ( SEb 0.64 ( 0.03 0.09 ( 0.01 0.31 ( 0.02 104 ( 2 For seed preparation, the glycerol frozen storage cells (OD ) 1) were slowly thawed, and 0.5 mL was a Experiment 5 is graphically shown in Figure 2, and experi- 500 nm ment 6 in Figure 3. b Standard error. c The elemental composition seeded into 100 mL of the medium. The initial glucose was estimated as CH1.72O0.47N0.24 (Andersson et al., 1996), hence concentration was 10 g/L. When the seed reached OD500 nm the biomass having 40 mmol of carbon per gram dry cell weight ) 4-5, the spent medium was removed by centrifugation, based on this formula. This was consistent with the study of and the cells were resuspended in a smaller volume of Andersen et al. (1980). d Total carbon recovered from biomass, fresh medium. This suspension was used as inoculum. acetate, and carbon dioxide as percentage of glucose carbon Analyses. Off-line enzymatic analyses of glucose, consumed. acetate, ethanol, formic acid, and lactate were performed using the kits provided by Boehringer Mannheim GMbH Medium and Culture Conditions. The defined (Mannheim, Germany) with the kit catalog numbers 716 mineral medium comprised the following (g/L): Na2SO4, 251, 148 261, 176 290, 979 732, and 1 112 821, respec- ‚ 2; (NH4)2SO4, 2.468; NH4Cl, 0.5; K2HPO4, 14.6; NaH2PO4 tively. The incubation during the enzymatic reactions and - H2O, 3.6; (NH4)2-H-citrate, 1; and thiamin, 0.01 0.1. the absorbance readings was performed on a semiauto- Three milliliters of MgSO4 (1 M) and trace element mated ELISA machine (Labsystem iEMS, Helsinki, solution were sterile-filtered (0.2 µm) into a 1-L auto- Finland), providing four repeated measurements for each claved medium. The trace element solution contained the sample. ‚ ‚ ‚ following (g/L): CaCl2 H2O, 0.5; ZnSO4 7H2O, 0.18; MnSO4 On-line gas composition was measured with a para- H2O, 0.1; Na2EDTA, 20.1; FeCl3‚6H2O, 16.7; CuSO4‚ ‚ magnetic oxygen analyzer (Sybron, Boston, MA) and an 5H2O, 0.16; and CoCl2 6H2O, 0.18. The initial batch infrared carbon dioxide analyzer (Leybold-Heraeus GmbH, glucose concentrations were varied from 4 to 14 g/L as Hanau, Germany). The exit gases were cooled and dried indicated in the figures and Table 1. The feeding solution through a silica column prior to analysis. Gas analysis had a glucose concentration of 500 g/L prior to analysis data were monitored with an eight-channel data acquisi- with double salt concentrations. All batch and fed-batch tion unit (PC-logger 2100, INTAB, Stenkullen, Sweden) cultivations were conducted in a 15-L stirred tank reactor which was connected to a PC (EM2 486) with the (Biostat E, Braun, Melsungen, Germany) if not otherwise Software PCLOG. The sampling frequency of the data indicated. As described in detail elsewhere (George, was 1 min-1. The specific rate of oxygen consumption, 1997), the reactor is equipped with controls for agitation, qO (mmol g-1 h-1), was obtained from the oxygen pH, temperature, and dissolved oxygen tension (DOT). consumption rate (OCR, mmol L-1 h-1) divided by the For pH control, 25% NH4OH was used. The DOT was biomass concentration. measured with a polarographic oxygen electrode (Ingold, For dry cell weight assay, the centrifugal test tubes Urdorf, Switzerland), and the signal was monitored with were predried to their constant weight.
Details
-
File Typepdf
-
Upload Time-
-
Content LanguagesEnglish
-
Upload UserAnonymous/Not logged-in
-
File Pages10 Page
-
File Size-