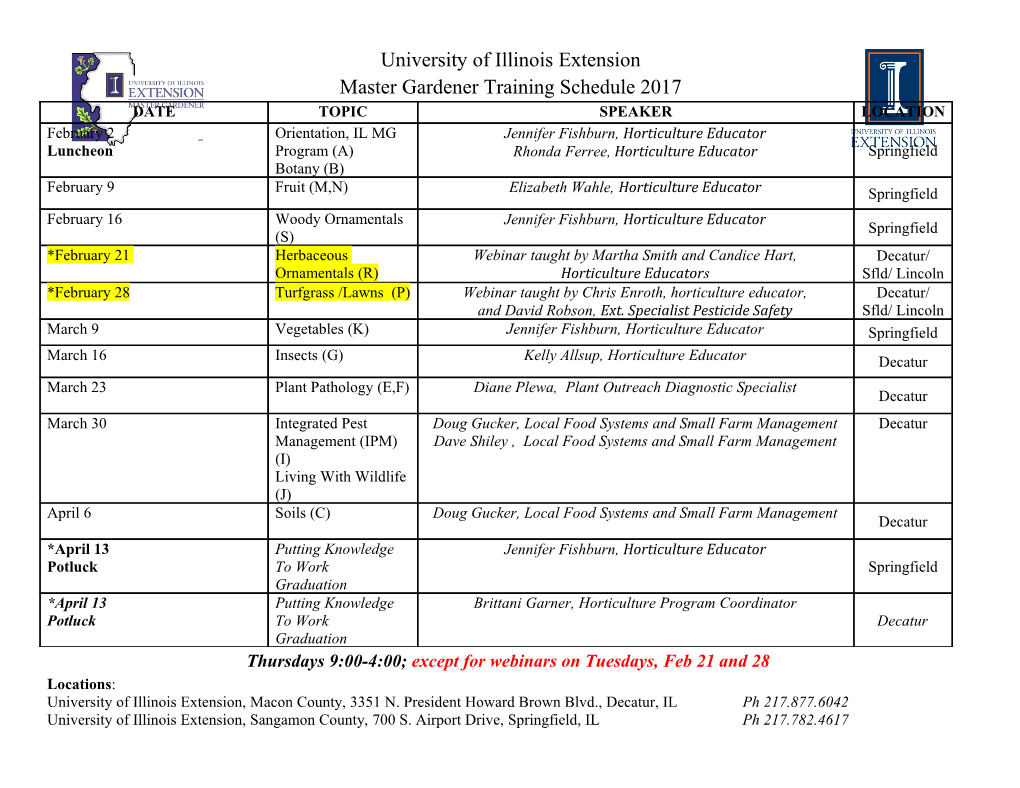
bioRxiv preprint doi: https://doi.org/10.1101/2020.03.15.992164; this version posted April 21, 2020. The copyright holder for this preprint (which was not certified by peer review) is the author/funder. All rights reserved. No reuse allowed without permission. Genome architecture and transcription data reveal allelic bias during the cell cycle Stephen Lindsly1, Wenlong Jia2, Haiming Chen1, Sijia Liu3, Scott Ronquist1, Can Chen4;7, Xingzhao Wen5, Gilbert Omenn1, Shuai Cheng Li2, Max Wicha1;6, Alnawaz Rehemtulla6, Lindsey Muir1, Indika Rajapakse1;7;∗ 1Department of Computational Medicine and Bioinformatics, University of Michigan, Ann Arbor 2Department of Computer Science, City University of Hong Kong 3MIT-IBM Watson AI Lab, IBM Research 4Department of Electrical Engineering and Computer Science, University of Michigan, Ann Arbor 5Department of Bioengineering, University of California San Diego, La Jolla 6Department of Hematology/Oncology, University of Michigan, Ann Arbor 7Department of Mathematics, University of Michigan, Ann Arbor ∗To whom correspondence should be addressed; E-mail: [email protected]. bioRxiv preprint doi: https://doi.org/10.1101/2020.03.15.992164; this version posted April 21, 2020. The copyright holder for this preprint (which was not certified by peer review) is the author/funder. All rights reserved. No reuse allowed without permission. Highlights • Structural and functional differences between the maternal and paternal genomes, includ- ing similar allelic bias in related subsets of genes. • Coupling between the dynamics of gene expression and genome architecture for specific alleles illuminates an allele-specific 4D Nucleome. • Introduction of a novel allele-specific phasing algorithm for genome architecture and a quantitative framework for integration of gene expression and genome architecture. Abstract Millions of genetic variants exist between paternal and maternal genomes in human cells, which result in unequal allelic contributions to gene transcrip- tion. We sought to understand differences in transcription and genome archi- tecture between the maternal and paternal genomes across the cell cycle. Our approach integrates haplotype-resolved genome-wide data from B-lymphocytes in G1, S, and G2/M, mature and nascent mRNA, genome wide chromosome conformation capture (Hi-C), and protein binding (ChIP-seq). Further, our novel method, HaploHiC, enables phasing of reads of unknown origin. Our data indicate significant allelic bias in cell identity genes, and notably absent bias in universally essential cell cycle and circadian rhythm genes. We offer a model in which coordinated biallelic expression reflects prioritized preserva- tion of essential gene sets. bioRxiv preprint doi: https://doi.org/10.1101/2020.03.15.992164; this version posted April 21, 2020. The copyright holder for this preprint (which was not certified by peer review) is the author/funder. All rights reserved. No reuse allowed without permission. Introduction Maternal and paternal chromosomes typically each encode an allele of the same gene. Mil- lions of single nucleotide variants (SNVs), insertions, and deletions (InDels) enable distinction between the maternal and paternal genome, and DNA sequence variations can influence 3D conformation of the genome (1). Studying the maternal and paternal alleles of a gene may en- hance our understanding of genome organization and mechanisms of bias in allele expression. Allelic differences have been observed through gene expression, 3D chromatin organization, and protein binding (2–9), and it has been shown that even subtle differences in genome struc- ture can lead to differential gene expression and regulation (10). Approximately 10-25% of all genes have allelic biased expression across many cell types (11–13). One well studied example of allele-specific expression and architecture is X chromosome inactivation (XCI) in females, in which one of the two X chromosomes is tightly packed into two ‘superdomains’ with silencing of gene expression (4, 14). Another example of known allele-specific expression is imprinting, in which one allele’s expression is suppressed (15). It has also been shown for single cells that monoallelic transcripts could make up over 75% of total transcripts (16). The allele-specific nature of genome architecture and gene expression have been explored individually, but it remains poorly understood how differences in chromosome architecture be- tween alleles contribute to differences in gene expression. To address this problem, we look to transcription factors and other regulatory proteins as key components that connect differences in genome conformation with differential expression of the maternal and paternal genomes. By integrating RNA sequencing (RNA-seq), bromouridine sequencing (Bru-seq), and genome- wide chromosome conformation capture (Hi-C), along with publicly available protein binding data (ChIP-seq), we contribute insight into how maternal and paternal genomes contribute to cell phenotype. The redundancy inherent in diploid genomes makes them robust to mutations and genetic loss-of-function events. In cancers, this redundancy is often missing, and referred to as loss of heterozygosity. For example, BRCA1 and BRCA2 exhibit loss of heterozygosity (17), and bioRxiv preprint doi: https://doi.org/10.1101/2020.03.15.992164; this version posted April 21, 2020. The copyright holder for this preprint (which was not certified by peer review) is the author/funder. All rights reserved. No reuse allowed without permission. mutations in these genes lead to a significant increase in the likelihood of developing breast cancer (18). Additionally, monoallelically expressed genes, like X-inactivated and imprinted genes (15, 19–21), do not have a ‘backup’ gene to compensate for potential deleterious muta- tions, and it has been found that mutations in monoallelicly expressed genes are responsible for disease phenotypes (22). These findings lend credence to the pursuit of allele-specific genome analysis. The 4D Nucleome (4DN) describes the relationship between genome architecture, gene expression, and cell phenotype through time (23). Chen et al. (24) introduced a mathematical framework for the 4DN, which can be applied in an allele-specific manner to a fully haplotyped cell line (e.g. NA12878). Despite extensive study of genome replication and compaction in cell division, few studies focus on differences between maternal and paternal genomes during these processes. For example, the two alleles may have different dynamics through the cell cycle that influence proliferation, or an allele-specific 4DN. In this paper, we integrate allele-specific RNA-seq, Bru-seq, Hi-C (Figure 1), and ChIP-seq data to provide a more comprehensive understanding of the maternal and paternal contributions to phenotype, and the relationship between these properties. We analyze differential expression for allele-biases and allele-specific cell cycle-biases, chromatin compartment switching (also known as A/B compartments), and Topologically Associated Domains (TADs). In addition, we analyze our Hi-C data using our recently developed methods that help discern differences in genome architecture between the two genomes and through the cell cycle. Our methods for analyses reveal notable patterns of allelic bias across the cell cycle, including subsets of genes involved in related cellular processes. This work supports the idea that separate analysis of the two parental genomes is imperative to future genomic studies. bioRxiv preprint doi: https://doi.org/10.1101/2020.03.15.992164; this version posted April 21, 2020. The copyright holder for this preprint (which was not certified by peer review) is the author/funder. All rights reserved. No reuse allowed without permission. Fig. 1: Experimental and allelic separation workflow. Cell cycle sorted cells were extracted for RNA-seq, Bru-seq, and Hi-C (left to right, respectively). RNA-seq and Bru-seq data were allelicly phased via SNVs/InDels (left). SNV/InDel based imputation and allelic phasing done using HaploHiC (right). bioRxiv preprint doi: https://doi.org/10.1101/2020.03.15.992164; this version posted April 21, 2020. The copyright holder for this preprint (which was not certified by peer review) is the author/funder. All rights reserved. No reuse allowed without permission. Results Chromosome level differences of the maternal and paternal genomes In order to establish whether there are allele-specific differences in both chromosome structure and gene expression, we analyzed parentally phased whole-chromosome Hi-C matrices and RNA-seq data at a 1 Mb scale (phasing discussed below, in Supplementary Notes 2.1.7, and 2.4). We performed a simple subtraction of the phased Hi-C matrices and found the Frobenius norm of the difference matrices, adjusted for chromosome size. Similarly, we subtracted the phased RNA-seq vectors and found the Frobenius norm of each difference vector, adjusted for chromosome size. If the two parents’ genomes were equivalent, the resulting chromosome-level Frobenius norms would approach zero. As seen in Figure 2, we found that all chromosomes have allelic differences in both structure (blue) and function (red). Unsurprisingly, chromosome X had the largest structural difference, followed by chromosomes 9, 21, and 14. The X chro- mosome also had the most extreme functional difference, followed by chromosomes 13, 7, and 9. We standardized the Frobenius norms of all chromosomes by the norm of the X chromo- some, as its value was much larger than any of the autosomes.
Details
-
File Typepdf
-
Upload Time-
-
Content LanguagesEnglish
-
Upload UserAnonymous/Not logged-in
-
File Pages57 Page
-
File Size-