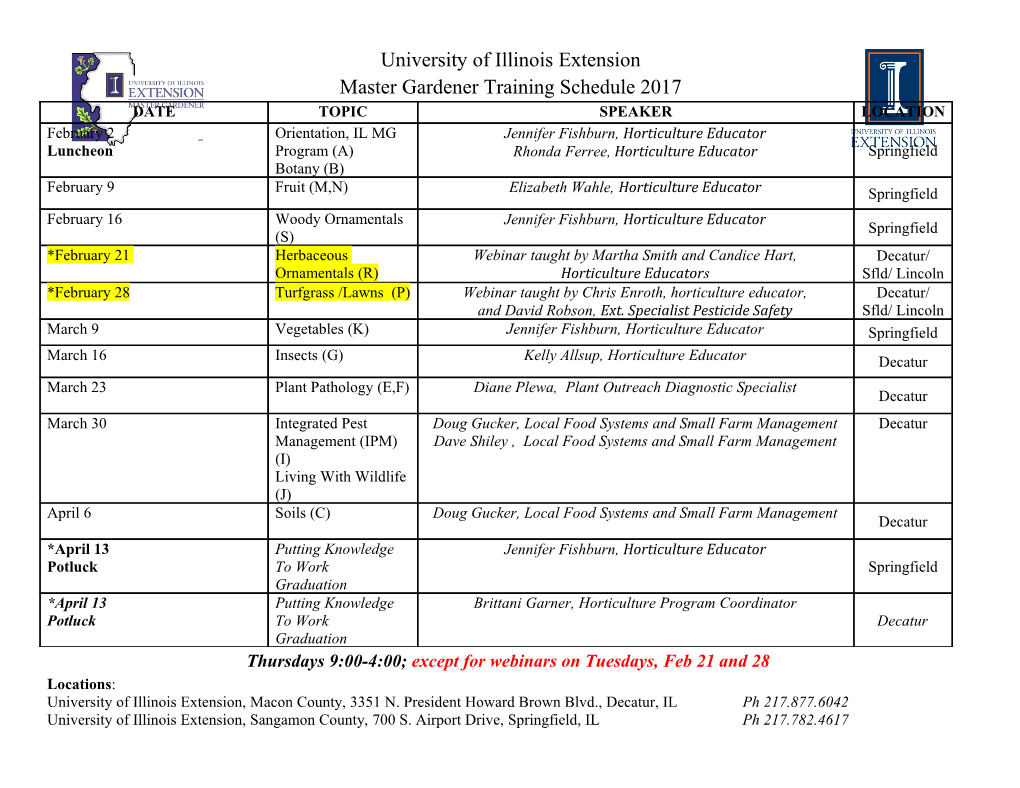
ERJ Express. Published on February 8, 2013 as doi: 10.1183/09031936.00078812 Application of ‘omics technologies to biomarker discovery in inflammatory lung diseases 1,2Craig E. Wheelock, 3Victoria M. Goss, 1,2David Balgoma, 3Ben Nicholas, 3Joost Brandsma, 4Paul J. Skipp, 1,2Stuart Snowden, 5Arnaldo D'Amico, 6Ildiko Horvath, 7Amphun Chaiboonchoe, 7Hassan Ahmed, 7Stéphane Ballereau, 8Christos Rossios, 8Kian Fan Chung, 9Paolo Montuschi, 10Stephen J. Fowler, 8Ian M. Adcock, 3Anthony D. Postle, 2,11Sven‐Erik Dahlén, 12Anthony Rowe, 13Peter J. Sterk, 7Charles Auffray, 3Ratko Djukanović and the U‐ BIOPRED Study Group 1Department of Medical Biochemistry and Biophysics, Division of Physiological Chemistry II, Karolinska Institutet, Stockholm, Sweden; 2Centre for Allergy Research, Karolinska Institutet, Stockholm, Sweden; 3Clinical and Experimental Sciences, University of Southampton Faculty of Medicine and NIHR Southampton Respiratory Biomedical Research Unit, Southampton, United Kingdom; 4Centre for Biological Sciences, University of Southampton Faculty of Natural and Environmental Sciences and Centre for Proteomic Research, Southampton, United Kingdom; 5Department of Electronic Engineering, University of Rome "Tor Vergata", Rome, Italy; 6Department of Pulmonology, Semmelweis University, Budapest Dios arok 1/C, H‐1125, Hungary; 7CNRS Institute of Biological Sciences, European Institute for Systems Biology & Medicine, Claude Bernard University, Lyon, France; 8Airways Disease, National Heart & Lung Institute, Imperial College London, United Kingdom; 9Department of Pharmacology, Faculty of Medicine, Catholic University of the Sacred Heart, Rome, Italy; 10Lecturer and Honorary Consultant in Respiratory Medicine, University of Manchester and Lancashire Teaching Hospitals NHS Foundation Trust, Respiratory Research Group, Education and Research Centre, Wythenshawe Hospital, Manchester, United Kingdom; 11Unit for Experimental Asthma and Allergy Research, Karolinska Institutet, Stockholm, Sweden; 12Janssen Research and Development Ltd, High Wycombe, UK 13Department of Respiratory Medicine, Academic Medical Center University of Amsterdam, Amsterdam, The Netherlands Address for correspondence: Ratko Djukanović, Clinical and Experimental Sciences, University of Southampton, and Southampton Respiratory Biomedical Research Unit, Mailpoint 810, Sir Henry Wellcome Laboratories, Level F, Southampton, University Hospitals Southampton NHS Foundation Trust, Southampton SO16 6YD, United Kingdom 1 Copyright 2013 by the European Respiratory Society. Abstract Inflammatory lung diseases are highly complex in respect of pathogenesis and relationships between inflammation, clinical disease and response to treatment. Sophisticated large‐scale analytical methods to quantify gene expression (transcriptomics), proteins (proteomics), lipids (lipidomics) and metabolites (metabolomics) in the lungs, blood and urine are now available to identify biomarkers that define disease in terms of combined clinical, physiological and patho‐biological abnormalities. The aspiration is that these approaches will improve diagnosis, i.e., define pathological phenotypes, and facilitate the monitoring of disease and therapy and, also, unravel underlying molecular pathways. Biomarker studies can either select pre‐defined biomarker(s) measured by specific methods or apply an “unbiased” approach involving detection platforms that are indiscriminate in focus. This article reviews the technologies presently available to study biomarkers of lung disease within the ‘omics field. The contributions of the individual ‘omics analytical platforms to the field of respiratory diseases are summarised, with the goal of providing background on their respective abilities to contribute to systems medicine‐based studies of lung disease. Key words: asthma, lung disease, transcriptomics, proteomics, lipidomics, metabolomics, systems biology, systems medicine 2 Introduction Inflammatory lung diseases are highly complex in respect of pathogenesis and relationships between inflammation, clinical disease and response to treatment. While interstitial lung diseases have long been viewed as a spectrum of distinct pathological conditions with different clinical outcomes (1), asthma and chronic obstructive pulmonary disease (COPD) have only recently been recognised as syndromes consisting of several disease entities (2‐6). Sophisticated, high‐throughput, large‐scale analytical methods to quantify gene expression, proteins and lipids as well as other metabolites in the lungs, blood and urine are now available. These methods offer the potential to identify biomarkers that define airways obstructive diseases in terms of combined clinical, physiological and patho‐biological abnormalities. The aspiration is that these approaches will improve diagnosis, i.e., define disease phenotypes and facilitate the monitoring of disease activity and therapy. In research terms, this information will also help unravel the complex molecular pathways underpinning disease. In broad terms, biomarker studies can either select pre‐defined biomarker(s) measured by specific methods or apply an “unbiased” approach involving use of indiscriminate detection platforms. This article reviews the technologies presently available to study biomarkers of lung disease within the so‐called ‘omics field, a term that was first used to define the studies of genomes (genomics) and gene expression (transcriptomics) of cells, tissues, organs and organisms and has subsequently been adopted for studies of proteins (proteomics), lipids (lipidomics) and metabolites (metabolomics). More recently, measurement of volatile organic compounds (VOCs) in exhaled breath condensate has been termed “breathomics”. The use ofe th ‘omics term reflects an experimental paradigm based upon the acquisition of large‐scale datasets from a single sample with the aim of identifying biomarkers of disease and/or elucidating novel functional or pathological mechanisms (Figure 1). An ‘omics experimental design often involves a hypothesis‐generating component in which a broad encompassing dataset is acquired to provide insight into novel processes in disease, rather than focusing on reductionist “molecular medicine‐based” targeted methodologies. ‘Omics approaches are resource‐intensive, analytically demanding and require the use of sophisticated statistical and modelling approaches to analyse datasets consisting of hundreds to thousands of variables in order to minimize false positives (Type I error) and false negatives (Type II error). The collection of ‘omics‐based datasets is often an integral component of systems biology studies, which seek to integrate data and thereby understand key fluctuations in the homeodynamics of the experimental system in question (i.e., disease, phenotype, therapeutic intervention). Regardless of the technique chosen, the diagnostic accuracy of potential identified biomarkers has to be examined and validated according to international recommendations based on STARD‐ guidelines (www.stard‐statement.org) (7). The principal biological matrices available for biomarker discovery in respiratory diseases are: whole lung tissue and cells isolated from lung parenchyma, bronchoalveolar lavage fluid (BALF), spontaneous or induced sputum, exhaled air, exhaled breath 3 condensate (EBC), blood (cells, serum and plasma), and urine. Their advantages and disadvantages for application in the various ‘omics platforms are summarized in Table 1, with a glossary of terms provided in Table 2. For example, lung tissue contains combined transcriptomes, proteomes and metabolomes of multiple cell types, which complicates analysis and data interpretation. By comparison, EBC may have a proteomic profile that is too simple to empower meaningful biomarker studies (8). Accordingly, it is important that the potential constraints of a given matrix are considered when evaluating method development for any of the ‘omics approaches discussed in this review. A key consideration for ‘omics methods is the wide dynamic range of analytes in biological samples (9, 10). This can result in ‘crowding out’ of less abundant analytes by more abundant ones, and as a consequence requires methodologies to enrich low abundance components, but at the price of adverse affects on assay reproducibility. This range in concentrations can also have repercussions for statistical modelling, (e.g., use of univariate scaling vs. no scaling of data, which both make very different assumptions regarding the biological significance of the data). Analysis is further complicated by the physico‐chemical diversity of some analytes, e.g., proteins due to alternative splicing, RNA editing and subunit oligomerisation and post‐translational modifications (11). Lipids have a wide diversity of structural and physical properties, ranging from neutral molecules, such as triacylglycerols and sterols, through polar glycerophospholipids, to signalling molecules such as eicosanoids and other oxylipins, which comprise numerous isomers. In addition, developed methodologies rarely consider stereochemistry in compound analysis and identification, which can have profound effects on observed biological parameters. This structural and physico‐chemical diversity, in combination with dynamic ranges of several orders of magnitude, results in an abundance of analytical challenges associated with each of the different ‘omics platforms that need to be considered in method development on a matrix‐specific basis. In addition, the variety in the acquired
Details
-
File Typepdf
-
Upload Time-
-
Content LanguagesEnglish
-
Upload UserAnonymous/Not logged-in
-
File Pages45 Page
-
File Size-