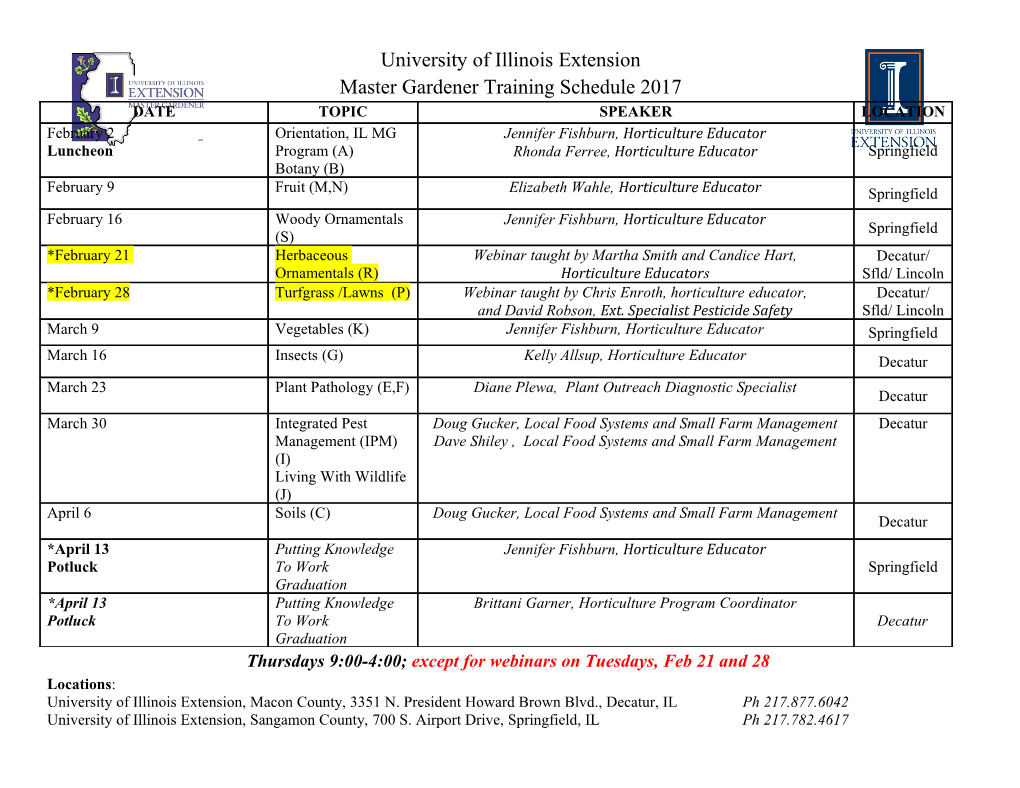
Dynamic evolution of translation initiation mechanisms in prokaryotes So Nakagawaa, Yoshihito Niimurab, Kin-ichiro Miurac,1, and Takashi Gojoboria,d,2 aCenter for Information Biology and DNA Data Bank of Japan, National Institute of Genetics, Mishima 411-8540, Japan; bDepartment of Bioinformatics, Medical Research Institute, Tokyo Medical and Dental University, Tokyo 113-8510, Japan; cDepartment of Medical Genome Science, Graduate School of Frontier Science, University of Tokyo, Kashiwa 277-8562, Japan; and dBiomedicinal Information Research Center, National Institute of Advanced Industrial Science and Technology, Tokyo 135-0064, Japan Communicated by Tomoko Ohta, National Institute of Genetics, Mishima, Japan, February 23, 2010 (received for review December 7, 2009) It is generally believed that prokaryotic translation is initiated by yotes, translation is generally initiated by a scanning mechanism. the interaction between the Shine-Dalgarno (SD) sequence in the The small (40S) ribosomal subunit, with several initiation factors, 5′ UTR of an mRNA and the anti-SD sequence in the 3′ end of a 16S binds the 7-methyl guanosine cap (11) at the 5′ end of an mRNA. ribosomal RNA. However, there are two exceptional mechanisms, It moves along the mRNA until it encounters an AUG codon which do not require the SD sequence for translation initiation: that is surrounded by a particular sequence such as the Kozak one is mediated by a ribosomal protein S1 (RPS1) and the other sequence (12). Hernández (13) hypothesized that the emergence used leaderless mRNA that lacks its 5′ UTR. To understand the of a nucleus led to the disappearance of the SD interaction and evolutionary changes of the mechanisms of translation initiation, establishment of other mechanisms of translation initiation in we examined how universal the SD sequence is as an effective eukaryotes. Thus, it appears that the mechanism of translation initiator for translation among prokaryotes. We identified the SD initiation in eubacteria and archaebacteria have not changed sequence from 277 species (249 eubacteria and 28 archaebacteria). during evolution as a result of the absence of a nucleus. We also devised an SD index that is a proportion of SD-containing However, two exceptional mechanisms of translation initiation genes in which the differences of GC contents are taken into have been identified in prokaryotes. One is translation initiation account. We found that the SD indices varied among prokaryotic mediated by a ribosomal protein S1 (RPS1), which is a component species, but were similar within each phylum. Although the anti- of the 30S ribosomal subunit. In Escherichia coli, RPS1 interacts SD sequence is conserved among species, loss of the SD sequence with a 5′ UTR of an mRNA, initiating translation efficiently, seems to have occurred multiple times, independently, in different regardless of the presence of the SD sequence (14, 15). RPS1 of E. phyla. For those phyla, RPS1-mediated or leaderless mRNA-used coli contains six S1 domains that are essential for RNA binding, mechanisms of translation initiation are considered to be working although the number of domains is different among species (16). to a greater extent. Moreover, we also found that some species, Recently, Salah and colleagues (17) analyzed the molecular such as Cyanobacteria, may acquire new mechanisms of transla- diversity of RPS1s, and classified them into four types depending tion initiation. Our findings indicate that, although translation ini- on their functional reliability of translation initiation, suggesting tiation is indispensable for all protein-coding genes in the genome that the function of RPS1 in translation initiation is different of every species, its mechanisms have dynamically changed among prokaryotes. during evolution. The other mechanism of translation initiation is for leaderless mRNAs that lack their 5′ UTR. A leaderless mRNA directly binds dynamic evolution | Shine-Dalgarno sequence | ribosomal protein S1 a 70S ribosome including an N-formyl-methionyl-transfer RNA, where translation is initiated (18–20). Leaderless mRNAs have ranslation initiation is fundamentally important for all pro- been found in various species of prokaryotes, particularly in arch- Ttein-coding genes in the genome of every organism. Ini- aebacteria (21–23). For example, in Halobacterium salinarum, tiation, rather than elongation, is usually the rate-limiting step in which belongs to the Euryarchaeota, leaderless mRNAs show a 15- translation, and proceeds at very different efficiencies depending fold higher activity in translation than mRNAs with the SD on the sequences in the 5′ UTRs of mRNAs (1). In prokaryotes sequence (24). This suggests that the SD interaction might not (for both eubacteria and archaebacteria), the Shine-Dalgarno necessarily be effective for translation initiation in some species (SD) sequence in an mRNA is well known as the initiator ele- of prokaryotes. Rather, the presence of these two mechanisms ment of translation (2, 3). The SD sequence, typically GGAGG, implies the possibility that the mechanisms of translation initiation is located approximately 10 nucleotides upstream of the initiator have diversified among prokaryotes (25). However, the evolu- codon. The SD sequence pairs with a complementary sequence tionary changes of these mechanisms of translation initiation (CCUCC) in the 3′ end of a 16S rRNA. In the 16S rRNA, the among prokaryotes are still unclear. In particular, it is interesting to sequence is called the anti-SD sequence in the 3′ tail of which know how universal the SD sequence is as the effective initiator for region is single-stranded. The interaction between the SD and translation among prokaryotes. the anti-SD sequences (called the SD interaction) augments The main purpose of this article is to answer this question to initiation by anchoring the small (30S) ribosomal subunit around understand the evolutionary processes of translation initiation. the initiation codon to form a preinitiation complex (4). The We examined the genomes of 277 prokaryotes belonging to 14 importance of the SD interaction for efficient initiation of phyla of eubacteria and three phyla of archaebacteria (Dataset S1). translation has been experimentally verified for both eubacteria and archaebacteria. Alterations of the SD sequence or the anti- SD sequence strongly inhibit protein synthesis, both in eubac- Author contributions: S.N., Y.N., K.-i.M., and T.G. designed research; S.N. performed re- teria including Escherichia coli (5) and Bacillus subtilis (6, 7) and search; S.N. contributed new reagents/analytic tools; S.N. analyzed data; and S.N., Y.N., in archaebacteria such as Methanocaldococcus jannaschii (8). For and T.G. wrote the paper. this reason, the SD interaction is thought to be the universal The authors declare no conflict of interest. mechanism of translation initiation in prokaryotes (9, 10). 1Deceased September 21, 2009. Although translation initiation is essential for all protein- 2To whom correspondence should be addressed. E-mail: [email protected]. coding genes in the genome of every species, its mechanisms are This article contains supporting information online at www.pnas.org/cgi/content/full/ quite different between prokaryotes and eukaryotes. In eukar- 1002036107/DCSupplemental. 6382–6387 | PNAS | April 6, 2010 | vol. 107 | no. 14 www.pnas.org/cgi/doi/10.1073/pnas.1002036107 Downloaded by guest on September 28, 2021 Our comparative analysis of a wide variety of genomes provides Table 1. Number of species and mean dRSD and RSD in the 17 a comprehensive picture of the evolution of the mechanisms of phyla used in this study translation initiation. Phylum No. of species dRSD RSD Results Prokaryotes 277 0.390 0.627 Conservation of the Anti-SD Sequence in 16S rRNA. To identify the Eubacteria 249 0.404 0.635 SD sequence in the genomes of 277 prokaryote species, we first Actinobacteria 22 0.383 0.673 determined the 3′ terminal sequence of a 16S rRNA, which Aquificae 1 0.358 0.515 includes the anti-SD sequence, in each species. We defined the last Bacteroidetes 4 −0.149 0.143 13 bases of a 16S rRNA as the 3′ tail sequence, which is the same Chlamydiae 7 0.361 0.541 as that of E. coli (GAUCACCUCCUUA). We found that the Chlorobi 3 0.095 0.338 annotated sequences of 16S rRNAs in 98 of the 277 species do not Chloroflexi 2 0.560 0.752 contain the nucleotide sequence CCUCC, the anti-SD sequence of Cyanobacteria 9 0.012 0.386 E. coli, in their 3′ tails. It is known that the annotation of 16S Deinococcus-Thermus 3 0.258 0.588 rRNAs is often dubious (26). Therefore, to determine whether the Firmicutes 51 0.636 0.770 absence of the anti-SD sequence is an annotation error, we Mollicutes 14 0.414 0.513 extended the terminal sequences of each of the 16S rRNAs using non-Mollicutes 37 0.720 0.868 the genome sequences of the 277 prokaryotes. We then con- Fusobacteria 1 0.607 0.697 structed a multiple alignment from these sequences. As a result, we Planctomycetes 1 0.086 0.335 identified a highly conserved motif in the extended 3′ end of 16S Proteobacteria 138 0.368 0.620 rRNAs of each species corresponding to the sequence of 3′ tail of a α 43 0.335 0.578 16S rRNA of E. coli (Fig. 1). In particular, the anti-SD sequence β 25 0.260 0.591 was completely conserved in all species examined, except for three δ 11 0.450 0.710 archaebacteria in which the cytosine at the last position is sub- ε 6 0.587 0.741 stituted by adenine. We also found several other highly conserved γ 53 0.405 0.636 motifs in the upstream region of the 3′ tail (Fig. S1 shows align- Spirochaetes 6 0.498 0.626 ment of 16S rRNAs). We therefore used the obtained 3′ tails of Thermotogae 1 0.543 0.871 16S rRNAs of each species for identification of the SD sequence Archaea 28 0.259 0.553 in this study.
Details
-
File Typepdf
-
Upload Time-
-
Content LanguagesEnglish
-
Upload UserAnonymous/Not logged-in
-
File Pages6 Page
-
File Size-