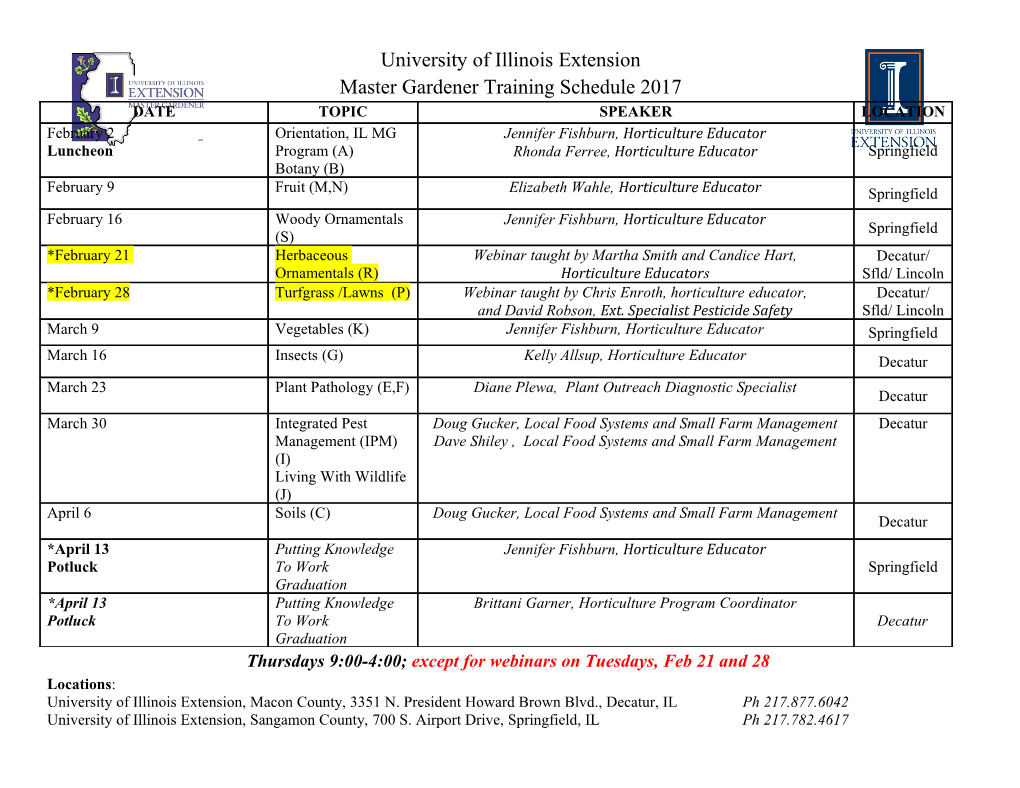
Signature of hydrophobic hydration SEE COMMENTARY in a single polymer Isaac T. S. Li and Gilbert C. Walker1 Department of Chemistry, University of Toronto, 80 St. George Street, Toronto, ON, Canada M5S 3H6 Edited by Robert Baldwin, Stanford University, Stanford, CA, and approved August 4, 2011 (received for review April 12, 2011) Hydrophobicity underpins self-assembly in many natural and syn- thetic molecular and nanoscale systems. A signature of hydro- phobicity is its temperature dependence. The first experimental evaluation of the temperature and size dependence of hydration free energy in a single hydrophobic polymer is reported, which tests key assumptions in models of hydrophobic interactions in protein folding. Herein, the hydration free energy required to ex- tend three hydrophobic polymers with differently sized aromatic side chains was directly measured by single molecule force spectro- scopy. The results are threefold. First, the hydration free energy per monomer is found to be strongly dependent on temperature and does not follow interfacial thermodynamics. Second, the temperature dependence profiles are distinct among the three hydrophobic polymers as a result of a hydrophobic size effect at the subnanometer scale. Third, the hydration free energy of a monomer on a macromolecule is different from a free monomer; corrections for the reduced hydration free energy due to hydro- CHEMISTRY phobic interaction from neighboring units are required. ydrophobic hydration involves the minimization of the free Henergies of water molecules near nonpolar surfaces. Hydro- phobic hydration of macromolecules is central to understanding Fig. 1. The role of hydrophobic collapse in the folding of three polymers protein folding (1–6) and advancing materials science and bio- with decreasing hydrophobicities: hydrophobic homopolymer, copolymer, technologies (7–9). A detailed methodology has been developed and protein (from top to bottom), plotting global free energy vs. radius of to study hydrophobic hydration by the mechanical unfolding of a gyration. Starting from an extended conformation in water, both protein collapsed single hydrophobic polymer (10). Typically, the hydra- and polymer chains are entropically reduced to random coils with reduced tion free energy (ΔGhyd) is assumed to scale with the solvent radii of gyration (red). When a random coil is compacted such that the accessible surface area (SASA) of molecules according to macro- chain can make frequent contact with itself, hydrophobic collapse (yellow) occurs to further reduce the global free energy and the radius of gyration. scopic interfacial thermodynamics, which is supported by the lin- If the hydrophobic units are sparser on the chain, hydrophobic collapse ear correlation between the SASA and the free energy to transfer occurs later during entropic coiling. For proteins, specific interactions (blue) hydrocarbons from a hydrophobic solvent (such as hexadecane) such as hydrogen bonds and salt bridges bring the protein to an energy to water (11). Despite a strong correlation, the experimentally minimum with the most compact structure. Hydrophobic polymers do not measured small hydrocarbon ΔGhyd is smaller than that predicted have such specific interactions and remain as compact random coils. by the calculated SASA (12), showing the breakdown of macro- scopic interfacial thermodynamics at the microscopic scale and Theoretical studies of hydrophobic hydration relied on experi- suggesting that the origin of the correlation goes beyond SASA. mental measurements of small molecule solubilities in water and In addition, the temperature dependence of ΔGhyd (the signature their transfer free energies from organic solvents to water. It is of hydrophobic hydration) varies according to the size of the usually assumed that these small molecule ΔGhyd can be directly solute; such temperature dependence cannot be explained simply applied to hydrophobic side chains in polymers and proteins. How- by the macroscopic interfacial free energy. In an earlier attempt ever, this assumption has not been experimentally tested due to the to address these issues, Tolman (13) developed a thermodynamic lack of a suitable experimental model system and the difficulty of treatment that lowers the surface tension of water at the solute- studying hydrophobic macromolecules insoluble in water. Hydro- water interface by taking into account the cavity curvature. Later phobic homopolymers provide simple models of the hydrophobic developments included temperature dependence of the Tolman hydration and collapse of proteins and other linear biological length using simulations to address the temperature dependence molecules (Fig. 1). Preceding hydrophobic collapse, an initially ex- of ΔGhyd (14, 15). However, it was argued that the correction to the effective surface tension to maintain the correlation between tended hydrophobic polymer in water undergoes entropic coiling ΔGhyd and SASA at small length scales lacked a clear physical meaning (16). Instead of depending on a scaling relation with Author contributions: I.T.S.L. and G.C.W. designed research; I.T.S.L. performed research; SASA, theories and simulations were developed predicting that G.C.W. contributed new reagents/analytic tools; I.T.S.L. and G.C.W. analyzed data; and ΔGhyd has nontrivial size dependence: Below approximately 1 nm I.T.S.L. and G.C.W. wrote the paper. radius, ΔGhyd of a spherical solute scales roughly with the solute The authors declare no conflict of interest. volume; whereas above this value, ΔGhyd scales with SASA and This article is a PNAS Direct Submission. asymptotically approaches the behavior described by macroscopic See Commentary on page 16491. interfacial thermodynamics (6, 17–28). These treatments cor- 1To whom correspondence should be addressed. E-mail: [email protected]. ΔGhyd rectly predict the trend for the temperature dependence of This article contains supporting information online at www.pnas.org/lookup/suppl/ for small molecules. doi:10.1073/pnas.1105450108/-/DCSupplemental. www.pnas.org/cgi/doi/10.1073/pnas.1105450108 PNAS ∣ October 4, 2011 ∣ vol. 108 ∣ no. 40 ∣ 16527–16532 Downloaded by guest on September 29, 2021 that reduces the tension along the chain to a point where the coil- that free monomer ΔGhyd cannot be directly applied to calculate globule transition can occur. The onset of this transition depends the hydration free energy of a hydrophobic macromolecule. on the overall hydrophobicity of the polymer such that the transi- tion begins at higher tension and radius of gyration for a hydro- Results phobic homopolymer than for a mixed hydrophobic/hydrophilic Single Polymer Force Plateau Marks Hydrophobic Hydration. Using polymer such as a protein (Fig. 1). Similar to a protein, a hydro- AFM, a single polymer chain can be pulled from a naturally phobic homopolymer collapses in an aqueous environment into a collapsed state to a hydrated, extended state as illustrated in compact globule that is largely disordered. This collapse captures Fig. 2A. It has been predicted theoretically and shown in simula- the essential physics governing the hydrophobic hydration of a tions that forced hydration of a collapsed hydrophobic polymer protein and is illustrated in Fig. 1. Ensemble studies of hydropho- produces a constant force-distance profile. (29–31) Force-exten- bic polymers are not viable because of their insolubility in water sion curves of single hydrophobic polymers such as polystyrene and limited experimental access to different conformational states (PS), poly(4-tert-butylstyrene) (PtBS), and poly(4-vinylbiphenyl) (e.g., extended and collapsed). However, by way of single molecule (PVBP) show characteristic constant force plateaus correspond- force spectroscopy using atomic force microscopy (AFM), the ing to single-chain hydration (10, 32–34), followed by entropic conformation of a single hydrophobic polymer can be changed elastic stretching before the chain detaches from the AFM can- from collapsed globule to extended coil (Fig. 2A)andΔGhyd in this B B tilever or the Si substrate (Fig. 2 ). Statistical analysis of the force process can be estimated (Fig. 2 ). A previous study provides a plateau magnitudes from thousands of independent molecular detailed description of the control experiments undertaken here pulling events at a given temperature for a given polymer shows for the mechanical unfolding of a collapsed single hydrophobic a quantized distribution (Fig. S1), which suggests that the lowest polymer in aqueous solvents (10). This report presents the effect of temperature on hydrophobic hydration of several polymers and force plateau results from hydration of a single polymer chain. Furthermore, when normalized to the same contour length, the how the size of side chains affects their temperature dependence. A The result shows that a phenomenological model using SASA and force curves can be superimposed on top of one another (Fig. 3 ), macroscopic interfacial thermodynamics cannot account for the showing that the polymer chains have the same persistence length observed temperature dependence of macromolecular hydropho- and that the physics behind the forced hydration is length inde- bic hydration. The observed temperature dependence shows the pendent within the range of polymer sizes accessible to our ex- turnover behavior typically seen in the hydration of small hydro- periment (hundreds of nanometers) The constant force profile phobic molecules. Moreover, individual monomers along the chain is
Details
-
File Typepdf
-
Upload Time-
-
Content LanguagesEnglish
-
Upload UserAnonymous/Not logged-in
-
File Pages6 Page
-
File Size-