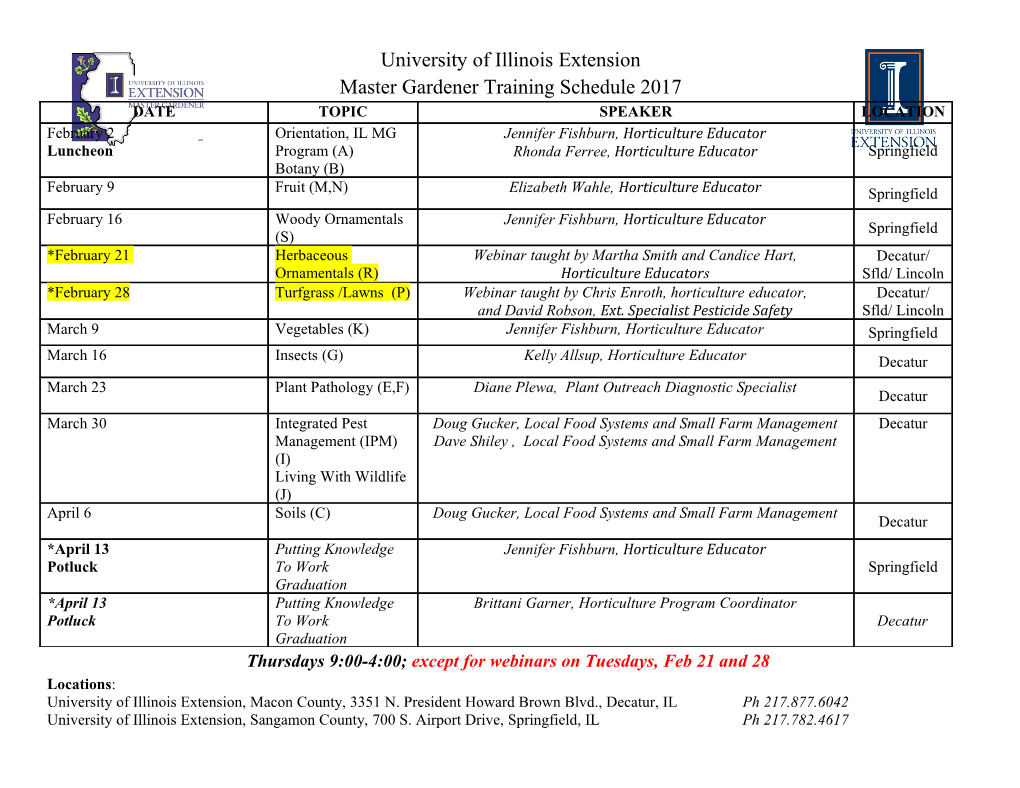
University of Nevada, Reno Optical Spectroscopy for Process Monitoring of 238Pu Production A thesis submitted in partial fulfillment of the requirements for the degree of Bachelor of Science in Materials Science and Engineering and the Honors Program by Aaron J. Unger Dr. Siddhartha Pathak/ Thesis Advisor Dr. Bin Li/ Thesis Advisor Dr. Kristian Myhre/ Thesis Advisor May, 2018 UNIVERSITY OF NEVADA THE HONORS PROGRAM RENO We recommend that the thesis prepared under our supervision by AARON J. UNGER entitled Optical Spectroscopy for Process Monitoring of 238Pu Production be accepted in partial fulfillment of the requirements for the degree of BACHELOR OF SCIENCE, MATERIALS SCIENCE AND ENGINEERING ______________________________________________ Bin Li, Ph.D., Thesis Advisor ______________________________________________ Tamara Valentine, Ph.D., Director, Honors Program May, 2018 TABLE OF CONTENTS SIGNATURE PAGE i CHAPTER I. ABSTRACT 1 II. INTRODUCTION AND BACKGROUND 3 a. Plutonium-238 Overview 3 b. Raman Spectroscopy 5 c. Laser-Induced Breakdown Spectroscopy 12 d. Remote Raman Spectroscopy Design 16 III. METHODS 18 IV. RESULTS AND DISCUSSION 21 a. Raman Spectroscopy 21 b. Determination of Optimal Thin Layer Chromatography Mobile Phase 27 c. Laser-Induced Breakdown Spectroscopy of Tributyl Phosphate on Alumina Plates 29 V. REMOTE RAMAN DESIGN 29 VI. CONCLUSION AND FUTURE WORK 36 APPENDIX A: Important Raman Modes 38 APPENDIX B: Thin Layer Chromatography Mobile Phase Compositions 40 REFERENCES 41 1 Optical Spectroscopy for Process Monitoring of 238Pu Production A. Unger I. Abstract 238Pu is a highly energetic isotope of plutonium recognized for its particularly high energy alpha particle emissions that can be easily attenuated to produce heat. The produced heat is then used for power production through radioisotope thermoelectric generators or radioisotope heater units which are commonly employed on spacecraft and probes. Manufacture of 238Pu has been sporadic in the United States, with isotope reserves decreasing rapidly. Oak Ridge National Laboratory’s High Flux Isotope Reactor has once again begun producing 238Pu for spacecraft and research applications with plans to scale to kilogram quantities per year. Fabrication of 238Pu involves processing of highly radioactive material that poses both proliferation, safety, and cost concerns. To ensure proper nuclear materials accountancy of 238Pu in its production, in situ species monitoring techniques must be developed. The efficacy of optical spectroscopic techniques have been studied to improve process monitoring capabilities of plutonium production. Raman spectroscopy and laser-induced breakdown spectroscopy calibration models have begun development for active determination of species concentrations in aqueous and organic streams. Raman spectroscopy is being investigated for use as an on-line, in situ process monitoring technique in 238Pu production due to its ability to probe samples rapidly and accurately. Deconvolution of process information using a nondestructive technique free of sample preparation will allow efficient processing of neptunium and plutonium and lowering of resource consumption. Design of experiment and multivariate analysis will be used to optimize calibration procedures for the Raman instrument in the system. While both organic and aqueous phases are of interest, focus has been placed on the aqueous modified direct denitrification (MDD) feed stream for this study. Species will primarily include nitrate, neptunyl, and plutonyl molecules. Measurements of single-component systems including nitric acid, sodium nitrate, and nitric acid containing concentrated cerium (as an initial neptunium surrogate) have been conducted allowing principal component analysis to elucidate important information regarding the nitrate species. Linear regression models were then compared to determine the repeatability of measurements on the basic systems. A fiber optic Raman system that can be used in a glovebox or hot cell for remote analysis has been designed and assessed, as well. Determination of phosphorus levels in the neptunium feed and plutonium product in the production of 238Pu is also crucial for optimizing process conditions and minimizing contamination of plutonium product. Spectroscopic and chromatographic techniques have been developed to produce models that can be used to quantify phosphorus levels and the degree of degradation of tributyl phosphate, an important extractant in the neptunium/ plutonium separation scheme. Laser-induced breakdown spectroscopy can be used to provide quantitative analysis of phosphorus contamination in unknown samples of material taken from the 238Pu production streams. Additionally, the degree of radiolysis of the tributyl phosphate extractant into its degradation products of dibutyl phosphate, monobutyl phosphate, and phosphoric acid can be determined by thin layer chromatography followed by laser- induced breakdown spectroscopy analysis of the separated species. Phosphorus in tributyl phosphate has been detected by laser-induced breakdown spectroscopy on samples of tributyl phosphate spotted onto alumina thin layer chromatography plates. Calibration sets that employ an internal 2 standard and are representative of the expected system will be developed and analyzed in a manner that minimizes variance among the system’s constituents. Thus, an accurate prediction model for quantification of the amount of contamination contributed by phosphorus containing species can be determined in a rapid and repeatable manner. 3 II. Introduction and Background Plutonium-238 Overview Plutonium-238 Applications The primary power source employed in many spacecraft and probes such as Voyager 1 is known as a radioisotope thermoelectric generator (RTG) which generates electrical energy by decay of radioisotopes. Attenuated radioactivity produces decay heat that is converted into electricity by the Seebeck effect using no moving parts and providing a long-term, reliable, and robust energy source. RTGs are generally powered by radioisotopes that demonstrate high specific power, have long half-lives, and emit easily absorbed radiation such as alpha particles. Long half-lives ensure that the power output remains relatively constant over the duration of the RTG’s mission and limits the isotopes that can be employed. 238Pu is an optimal isotope for RTG sources as it decays with a 5.593 MeV alpha particle over a half-life of 87.7 years resulting in a high power density of 0.57 W/g and is consequentially the most commonly used RTG power source 1. 238 A typical RTG requires approximately 3.5 to 8 kilograms of Pu creating 120 to 210 We of power respectively 1. The United States, however, has not produced the isotope since 1988 when the Savannah River Site closed and has since been purchasing the source from Russia, who has also recently shut down 238Pu production. The United States is now left with approximately 35 kilograms of reserves which will last NASA approximately two more years according to their projected project requirements as illustrated in Fig. 1. The figure also illustrates the need to continue production to meet the demands of planned science and exploration missions. As a result, the Department of Energy has decided to restart the 238Pu production program using the High Flux Isotope Reactor (HFIR) at Oak Ridge National Laboratory (ORNL) with plans to scale to about 5 kilograms of plutonium per year 1-3. Domestic fabrication of plutonium will enable future space missions of science and exploration using 238Pu based RTGs that are not possible without a non- Russian based source of 238Pu. Figure 1: NASA projected consumption of plutonium-238 through 2028 based on current mission design specifications. 1 Plutonium-238 Production The 238Pu isotope is formed by transmutation of 237Np followed by chemical processing to isolate the plutonium. 237Np is first dissolved in acid where it is further purified and decay products are 4 removed. The species is then converted to an oxide form as NpO2 and is blended with aluminum metal powder which then gets pressed into Np/Al pellets and loaded into an aluminum clad. These samples are known as the irradiation targets and are loaded into HFIR 1. The irradiation by high neutron flux stimulates the nuclear reaction that results in the 238Pu isotope through the two-step decay chain given in Equation 1 below. 237 238 93푁푝 + 푛 → 93푁푝 238 − 238 93푁푝 + 훽 → 94푃푢 푡1/2 = 2.103 푑 [4] 퐸푞푛. 1 Following irradiation, the targets rest and cool while the short-lived fission products and activation products decay. The targets are then dissolved in a caustic solution of NaOH and NaNO3 to separate the heavy metal oxides from the aluminum clad and matrix material waste. The heavy metal oxides are composed primarily of neptunium oxides and plutonium oxides but also contain residual fission and activation impurities such as thorium oxide 1,5. The oxides are recovered via filtration and dissolved in approximately 8M HNO3. The resulting solution is used as a feed for one of two potential routes of plutonium recovery: anion-exchange resin or solvent extraction. Anion-exchange is the conventionally used and developed route. The anion exchange method requires careful monitoring and manipulation of the nitric acid concentration and neptunium and plutonium oxidation states throughout interaction with the anion exchange column. Ferrous sulfamate (Fe(NH2SO3)2) is first added to reduce Np(V) and (VI) to Np(IV) while concurrently reducing Pu(VI) to Pu(III).
Details
-
File Typepdf
-
Upload Time-
-
Content LanguagesEnglish
-
Upload UserAnonymous/Not logged-in
-
File Pages46 Page
-
File Size-