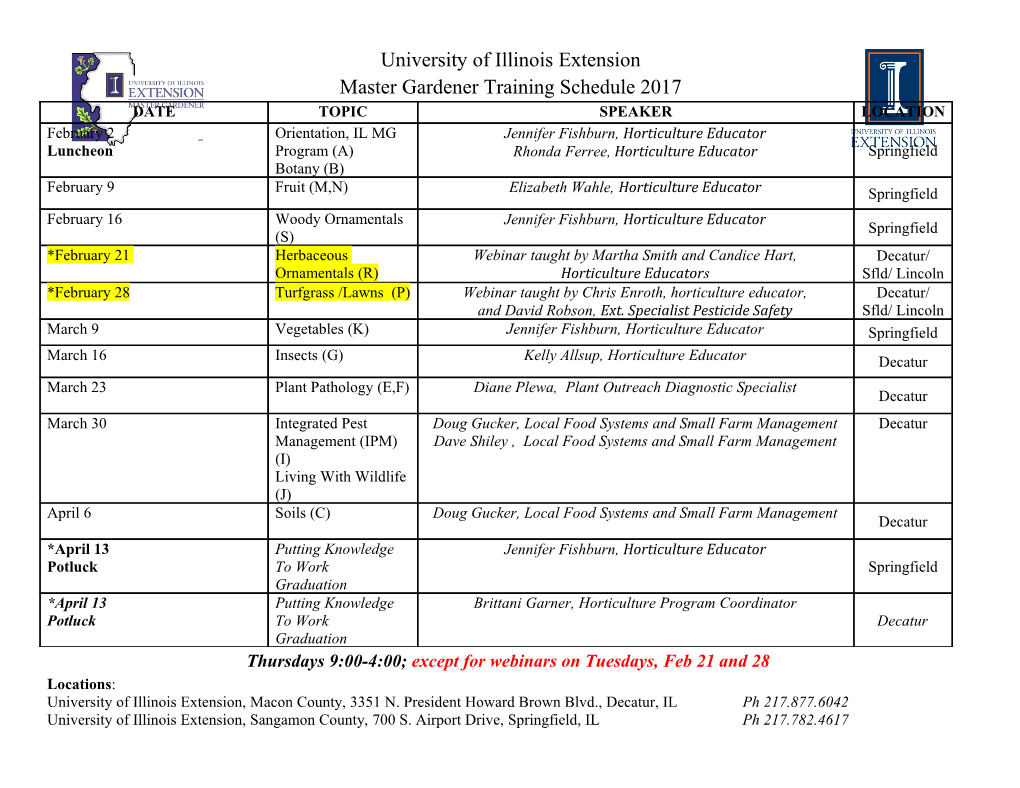
Thermodynamic Definitions System: A collection of material, or region in space, set aside for thermodynamic investigation. The system is chosen by the investigator. Boundary: Surface or surfaces, real or imaginary, by which either a closed or open system is delineated from the surroundings for analysis. Surrounding(Environment): Material, or regions in space, outside the boundaries of the system(s) but which may interact and influence system behavior. Closed system: A system that does not exchange mass with its surroundings. Open system: A system that exchanges mass with its surroundings. Isolated system: A system that does not interact with the environment. Changes of conditions in the environment have no influence on the behavior of isolated systems. Adiabatic system: A system that has no thermal interaction between system and surroundings. In practice, a thick layer of insulation material approximates an adiabatic wall. The adiabatic system may experience interactions other than thermal. Property: A property is a macroscopic characteristic of the system (mass, volume, energy, pressure, temperature, specific volume). A certain quantity is a property if, and only if, its change between two states is independent of the process (history). Thermodynamic property: A particular property from among the larger set on the basis of experiment and experience, has been found to be important for thermodynamic analysis. Extensive property: One whose numerical value depends on the extent of the system. In a homogeneous system, it is proportional to the mass of the system, e.g. volume, area, length, energy. Intensive property: One whose numerical value is independent of the extent of the system, e.g. density, pressure, stress, temperature. Temperature: The property of a system that determines whether or not it is in thermal equilibrium with another system and measures the hotness of the system as a number. Energy: A quantity that has the capacity for producing a change of state in a closed system. Work: A form of energy transfer. Work occurs when a force acts over a displacement. Heat Transfer: Another form of energy transfer. The energy transferred between systems, or between a system and it’s surrounding, due to a temperature difference. Dimension: An independent quantity that describes a system, a property of a system, or is necessary in the analysis of a system. e.g. time, length, area, volume, mass, temperature, force, energy. Thermodynamic state: The condition or circumstances of a system as described by values of its thermodynamic properties. The minimum set of independent thermodynamic properties sufficient to describe the state of the system is sometimes called the thermodynamic coordinates. Equilibrium: A state of affairs usually characterized by stability or the absence of tendency for spontaneous change. Systems which, upon isolation, are observed to exhibit no change in properties (and therefore state) are said to be in equilibrium. Thermodynamic equilibrium: The condition of a system such that all influences (thermal, mechanical, chemical, etc.) which have the potential for altering its state are in balance. Thermal equilibrium: A condition said to exist within a system or between a system and its surroundings, or between two systems, when, despite intimate thermal contact, no change in properties is observed. Mechanical equilibrium: No net force exists on or in the system acting from the surroundings or from within the system. Furthermore, no work transfers are occuring within subsystems and all parts of the system are at rest relative to each other and the surroundings Process: This describes the continuous series of state changes undergone by a system due to interactions between the system and another system, between the system and its surroundings, or due to an initial lack of equilibrium in the system that caused it to change spontaneously. Path: The locus of states through which a system passes when going from an initial state to a final state. For some systems, the thermodynamic state is described by only two independent properties. Hence, the path is a trace on a plane. Adiabatic process: A process in which no heat is exchanged with the environment. Cycle: A process, or series of processes, in which the initial and final states are identical. Laws: Experimental observations on systems that have been formulated into mathematical statements. Zeroth law of thermodynamics: When each of two subsystems is in thermal equilibrium with a third subsystem (i.e. thermometer), they are in thermal equilibrium with each other. First law of thermodynamics: The value of the net work done by or on a closed system undergoing an adiabatic process between two given states depends only on the end states and not on the details of the adiabatic process. (Energy conservation statement). The change in the total energy of a closed system during a process is equal to the heat transfer to the system minus the work done by the system. * Second law of thermodynamics: (Clausius statement) It is impossible for any system to operate in such a way that the sole result would be an energy transfer by heat from a cooler to a hotter body. (Kelvin-Planck statement) It is impossible for any system to operate in a thermodynamic cycle and deliver a net amount of work to its surroundings while receiving energy by heat transfer from a single thermal reservoir.( principle of work for the refireginator ?) Macroscopic thermodynamics: (classical thermodynamics) The continuum approximation holds so that the behavior of the system can be represented using observations on the system as a whole. The properties of a system are defined at a point rather than being averaged over the system. This course will be concerned only with classical thermodynamics. Microscopic thermodynamics: (statistical thermodynamics) The continuum approximation breaks down. Statistical methods are necessary to predict an average or macroscopic behavior of the system. Mean free path (λ): The average distance a molecule moves within a gas at a constant speed along a straight line between successive collisions. Thermodynamic Systems A “thermodynamic system” is a part of the physical world constituted by a significantly large number of particles (i.e., atoms or molecules)*. Examples are the gases, liquids, and solids, and their mixtures. The system is divided by what is called the “external ambient” or the “surroundings” by means of a well-defined boundary surface. These boundaries can be permeated or not by streams of mass, heat, and work. If any streams are not allowed, then the system is called “isolated.”. while the boundary surfaces are open to the exchange of mass, heat, and work with the surroundings. A system like this is called “open.” Generally, the features of the walls surrounding the systems define the constraints existing on the system. Thus, a wall that inhibits or allows the exchange of heat is called “adiabatic” or “diathermal,” respectively, while one that inhibits the exchange of mechanical work is called “rigid.” A classification of the thermodynamic systems as a function of their interaction with their surroundings is summarized in Table 1. System type Exchange Exchange Exchange of of mass of heat work Open Yes Yes Yes Close No Yes Yes Thermally No No Yes Isolated No No No Table 1. Classification of thermodynamic system The state of a system is completely defined from a mechanical point of view when the position and the velocity of any mass point of the system are known at a given instant of time. Thus, for a system formed by a number NP of mass points, 6NP variables should be known (i.e., three velocity components and three coordinates). the macroscopic properties of the system are defined as the “state of the system.” These macroscopic properties are defined through a series of measurable parameters or functions called “state functions” or “state variables.” For a given amount of the substance contained in the system, temperature, volume, and pressure are not independent one from the another. They are connected by a relationship of the general form Φ(P,V,T) = 0 which is called the “equation of state.” Generally, the form of the equation of state depends on the properties of the considered substance. Examples of equation of states are the ideal gas law, the Van der Waals equation for real gases and liquids.(exam) The Principle of Conservation of Energy In its most general form, the Principle of Conservation of Energy says that energy is “conserved”—that is, it can’t be created or destroyed, so that the total amount of energy in any closed system (that is, a system that can’t exchange energy with anything outside the system) doesn’t change. However, energy exists in many forms, and it can change from one form to another. For example, when an object emits radiative energy (which most objects do, all the time), that energy must come at the expense of some other form of energy, and it in fact comes at the expense of an equivalent amount of heat energy in the emitting substance. (Heat is a form of internal energy associated with the random molecular motions of a substance). Similarly, when an object absorbs radiative energy, that energy is converted into an equivalent amount of heat in the object. As another example, when a substance changes.phase (from gas, liquid, or solid to one of the other two phases), energy transforms from heat in the substance to an equivalent amount of latent heat (a form of chemical potential energy) in the substance, or vice versa, depending on the direction of the phase change. Potential Energy Potential energy is energy associated with the relative positions of two or more interacting particles. Potential energy fits well the idea of energy as the capacity to do work, e.g. gravitational and elastic potential energy Conservative Forces The work done by a conservative force [WAB = WAB] is independent of the path taken.
Details
-
File Typepdf
-
Upload Time-
-
Content LanguagesEnglish
-
Upload UserAnonymous/Not logged-in
-
File Pages9 Page
-
File Size-