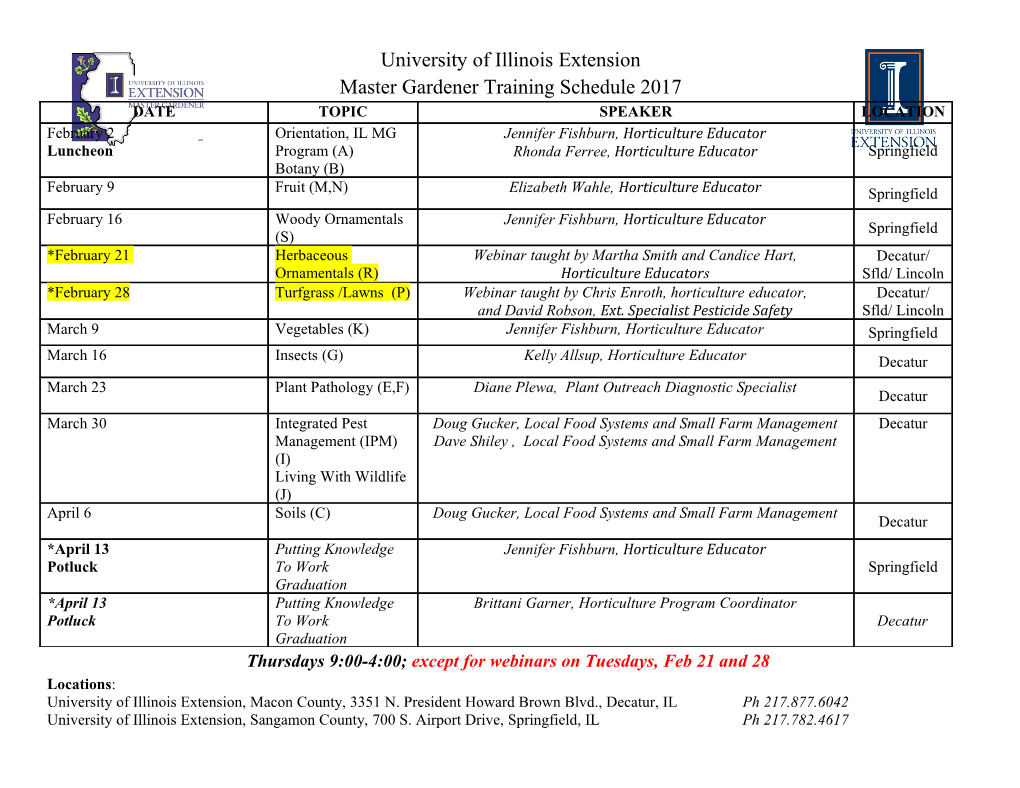
Author’s Accepted Manuscript Sources, variability and fate of freshwater in the Bellingshausen Sea, Antarctica Heather C. Regan, Paul R. Holland, Michael P. Meredith, Jennifer Pike www.elsevier.com PII: S0967-0637(17)30042-0 DOI: https://doi.org/10.1016/j.dsr.2018.01.005 Reference: DSRI2874 To appear in: Deep-Sea Research Part I Received date: 31 January 2017 Revised date: 15 January 2018 Accepted date: 16 January 2018 Cite this article as: Heather C. Regan, Paul R. Holland, Michael P. Meredith and Jennifer Pike, Sources, variability and fate of freshwater in the Bellingshausen Sea, Antarctica, Deep-Sea Research Part I, https://doi.org/10.1016/j.dsr.2018.01.005 This is a PDF file of an unedited manuscript that has been accepted for publication. As a service to our customers we are providing this early version of the manuscript. The manuscript will undergo copyediting, typesetting, and review of the resulting galley proof before it is published in its final citable form. Please note that during the production process errors may be discovered which could affect the content, and all legal disclaimers that apply to the journal pertain. Sources, variability and fate of freshwater in the Bellingshausen Sea, Antarctica Heather C. Regana,b, Paul R. Hollanda, Michael P. Mereditha, Jennifer Pikeb aBritish Antarctic Survey, Cambridge, United Kingdom. bSchool of Earth and Ocean Sciences, Cardiff University, United Kingdom. Abstract During the second half of the twentieth century, the Antarctic Peninsula was subjected to a rapid increase in air temperatures. This was accompanied by a reduction in sea ice extent, increased precipitation and a dramatic retreat of glaciers associated with an increase in heat flux from deep ocean water masses. Isotopic tracers have been used previously to investigate the relative importance of the different freshwater sources to the adjacent Bellingshausen Sea (BS), but the data coverage is strongly biased toward summer. Here we use a regional model to investigate the ocean's response to the observed changes in its different freshwater inputs (sea ice melt/freeze, precipitation, evaporation, iceberg/glacier melt, and ice shelf melt). The model success- fully recreates BS water masses and performs well against available freshwa- ter data. By tracing the sources and pathways of the individual components of the freshwater budget, we find that sea ice dominates seasonal changes in the total freshwater content and flux, but all sources make a comparable contribution to the annual-mean. Interannual variability is dominated by sea ice and precipitation. Decadal trends in the salinity and stratification of the ocean are investigated, and a 20-year surface freshening from 1992-2011 Preprint submitted to DSRI January 19, 2018 is found to be predominantly driven by decreasing autumn sea ice growth. These findings will help to elucidate the role of freshwater in driving cir- culation and water column structure changes in this climatically-sensitive region. Keywords: Bellingshausen Sea, Antarctica, Freshwater, Tracers, Sea ice trends 1 1. Introduction 2 From the 1950s until the late 1990s the Antarctic Peninsula (AP) warmed 3 more rapidly than any other region in the Southern Hemisphere, with air tem- ◦ 4 peratures increasing by nearly 3 C, though recent changes in wind patterns 5 may have led to a pause of the warming (Turner et al., 2016). Over the 6 same period, the summer surface ocean in the adjacent Bellingshausen Sea 7 (BS) warmed and salinified (Meredith and King, 2005). Unlike elsewhere 8 in Antarctica, the Bellingshausen and Amundsen seas have seen an overall 9 decrease in sea ice duration (Stammerjohn et al., 2012) and extent (Parkin- 10 son and Cavalieri, 2012) over the satellite era, with changes focussed on the 11 summer (Holland, 2014). Furthermore, along the AP, 87% of glaciers have 12 retreated since records began (Cook et al., 2005), with mass loss (Wouters 13 et al., 2015) and thinning (Paolo et al., 2015) observed in the southern BS 14 ice shelves. While atmospheric circulation changes and warming are thought 15 to be drivers, they cannot fully explain the ice loss, and recent indications 16 are that the ocean is playing an important role (Wouters et al., 2015; Cook 17 et al., 2016). 18 The BS can be characterised as being comprised of three water masses. 2 19 Below the permanent pycnocline, which is around 150-200 m on the shelf, 20 intrusions of Circumpolar Deep Water (CDW) from the Antarctic Circum- 21 polar Current (ACC) onto the shelf provide a source of heat and salt, with 22 the onshelf flow being especially effective within glacially-scoured canyons 23 that cross the shelf (e.g. Zhang et al. (2016), Klinck et al. (2004), Graham 24 et al. (2016)). In the northern BS the CDW layer has thickened and warmed 25 in recent decades (Martinson et al., 2008). This deep layer is overlain by 26 cool, fresh Antarctic Surface Water (AASW), which forms a homogeneous 27 layer around 50-150 m thick in winter, but which is capped in summer by a 28 relatively thin layer that is warmed by insolation and freshened by diverse 29 freshwater inputs. The subsurface temperature minimum that is created re- 30 flects the previous winter's mixed layer, and hence is termed Winter Water 31 (WW) (Klinck et al., 2004). 32 The freshwater balance of the BS is important because salinity controls 33 density in polar waters as thermal effects on density are small (e.g. Tal- 34 ley (2011), chapter 3), and therefore strongly affects ocean circulation and 35 mixing. It has been argued that cyclonic circulation on the shelf is ampli- 36 fied by freshwater-induced buoyancy effects (Savidge and Amft, 2009), and a 37 summer coastal current on the BS shelf is driven at least partially by glacial 38 melt and precipitation (Moffat et al., 2008). Sea ice melting and freezing, 39 and freshwater from meteoric sources (precipitation and evaporation, and 40 the melting of ice shelves, icebergs, and glacier fronts) may all contribute 41 significantly to the mean freshwater balance of the BS and its seasonality. 42 Increases in both precipitation days (Turner et al., 2005b) and snowfall 43 accumulation over longer timescales (Thomas et al., 2008) to the Antarctic 3 44 Peninsula suggest an increase in precipitation freshwater, particularly since 45 1950. This, along with the extensive retreat of glaciers in recent decades are 46 concurrent with increased calving ice and surface freshwater input into the 47 ocean. The potential consequences range from seasonal effects altering ocean 48 currents and stratification in summer, to influencing the formation of sea ice 49 in winter via surface ocean temperature changes and snow flooding. Sea ice 50 production may be enhanced by an increase in stratification that reduces 51 the oceanic heat flux from below (Hellmer, 2004). There are also important 52 biological consequences, as more glacial meltwater can enhance water column 53 stability and nutrient provision, favouring phytoplankton blooms (Dierssen 54 et al., 2002). 55 Basal melting of ice shelves varies significantly due to changes in the CDW 56 layer and wind strength (Holland et al., 2010; Dinniman et al., 2012), but 57 appears to have increased overall in the BS region (Paolo et al., 2015; Wouters 58 et al., 2015), causing ice-shelf thinning and increased meltwater input into the 59 ocean. This can cause numerous feedbacks, including stabilisation (Hellmer, 60 2004) or destabilisation (Merino et al., 2016) of the water column depending 61 upon the depth of meltwater injection, and intensification of coastal currents 62 (Nakayama et al., 2014). 63 The reduction in BS sea ice extent and duration, with an increased spring 64 meltwater flux (Holland, 2014), has a variety of effects. Reduced summer sea 65 ice cover can increase autumn ice production rates by exposing a greater area 66 of surface water to the atmosphere (Meredith et al., 2010). It can also change 67 basal melt rates of ice shelves (Holland et al., 2010) by altering stratification 68 and therefore the vertical flux of heat from CDW through the water column. 4 69 Given the strong climatic changes in the BS region in recent years, there is 70 a need to better understand the functional response of the different freshwa- 71 ter components to changing forcings so that their individual and collective 72 impacts on circulation, climate and the ecosystem can be determined and 73 better predicted. 74 There are a number of observations available to assist in closing the fresh- 75 water budget, though whilst spatial and temporal coverage is more complete 76 here than in any other region of the Southern Ocean, it is still strongly bi- 77 ased toward the summer season. In combination with salinity measurements, 18 78 oxygen isotope (δ O) measurements can separate meteoric freshwater inputs 79 from sea ice melt (Meredith et al., 2008), though further deducing contribu- 80 tions from each meteoric sink and source is not possible by this method. 81 Measurements in the northern BS show a general dominance of meteoric 82 water in coastal areas, though years of weak precipitation and/or extreme 83 sea ice can show comparable quantities of sea ice melt (Meredith et al., 84 2016). Over time there has been a decline in meteoric water in the surface 85 waters adjacent to Adelaide Island, north of Marguerite Bay, due to deep- 86 ening winter mixed layers (Meredith et al., 2013). This is despite increased 87 glacial discharge (Pritchard and Vaughan, 2007) and snowfall (Thomas et al., 88 2008) in the BS. However, interannual variability in freshwater inputs from 89 different sources and strong regional structure in their injection locations 90 can complicate the interpretation of data on wider temporal and spatial 91 scales (Meredith et al., 2016), highlighting the importance of understanding 92 the three-dimensional spatial variance of freshwater composition over time.
Details
-
File Typepdf
-
Upload Time-
-
Content LanguagesEnglish
-
Upload UserAnonymous/Not logged-in
-
File Pages58 Page
-
File Size-