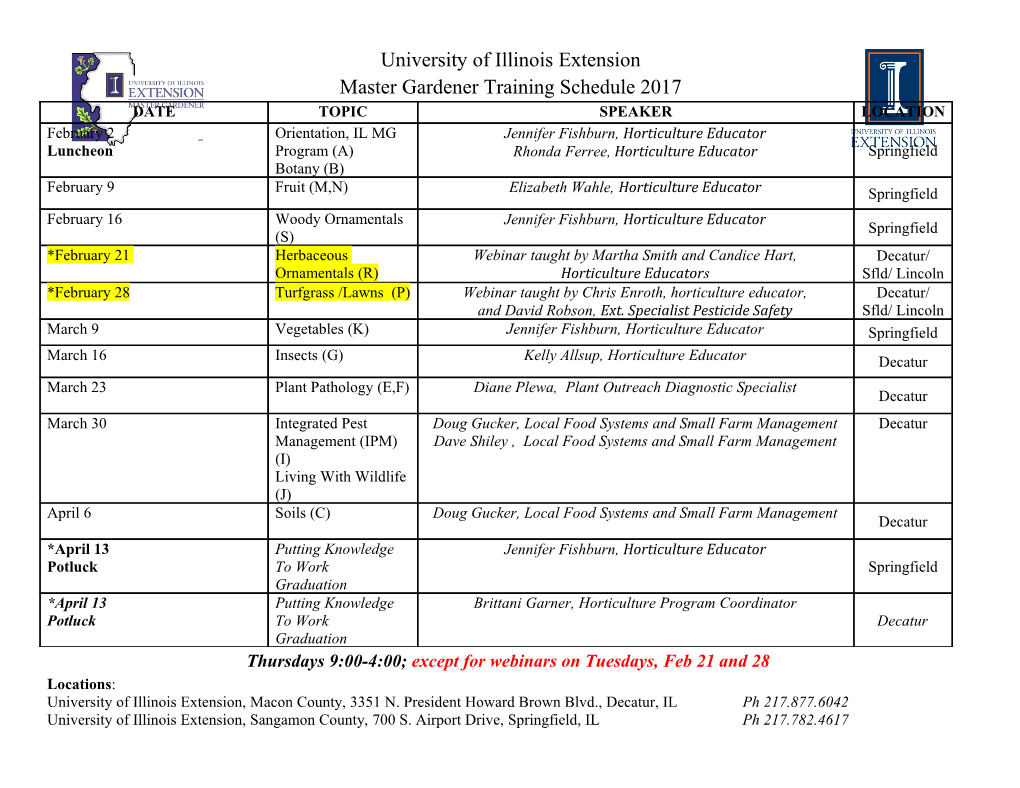
Lectures on Symplectic Geometry Fraydoun Rezakhanlou Departmet of Mathematics, UC Berkeley September 18, 2018 Chapter 1: Introduction Chapter 2: Quadratic Hamiltonians and Linear Symplectic Geometry Chapter 3: Symplectic Manifolds and Darboux's Theorem Chapter 4: Contact Manifolds and Weinstein Conjecture Chapter 5: Variational Principle and Convex Hamiltonian Chapter 6: Capacites and Their Applications Chapter 7: Hofer-Zehnder Capacity Chapter 8: Hofer Geometry Chapter 9: Generating Function, Twist Map and Arnold's Conjecture Chapter 10: Holomorphic Curves and Gromov's Width Appendix: A: Vector Fields and Differential Forms B: A Sobolev Inequality C: Degree Theory D: Cauchy and Beurling Transforms E: Lusternik-Schnirelmann Theory F: Morse Theory 1 1 Introduction Hamiltonian systems appear in conservative problems of mechanics as in celestial mechanics but also in statistical mechanics governing the motion of particles and molecules in fluid. A mechanical system of N planets (particles) is modeled by a Hamiltonian function H(x) d d where x = (q; p), q = (q1; : : : ; qN ), p = (p1; : : : ; pN ) with (qi; pi) 2 R × R being the position and the momentum of the i-th particle. The Hamiltonian's equations of motion are (1.1)q _ = Hp(q; p); p_ = −Hq(q; p); which is of the form ¯ ¯ 0 In (1.2)x _ = JrxH(x); J = ; −In 0 where n = dN and In denotes the n × n identity matrix. The equation (1.2) is an ODE that possesses a unique solution for every initial data x0 provided that we make some standard assumptions on H. If we denote such a solution by φt(x0) = φ(t; x0), then φ enjoys the group property φt ◦ φs = φt+s; t; s 2 R: The ODE (1.1) is a system of 2n = 2dN unknowns. Such a typically large system can not be solved explicitly. A reduction of such a system is desirable and this can be achieved if we can find some conservation laws associated with our system. To find such conservation laws systematically, let us look at a general ODE of the form dx (1.3) = b(x); dt with the corresponding flow denoted by φt, and study u(x; t) = Ttf(x) = f(φt(x)). A celebrated theorem of Liouville asserts that the function u satisfies @u (1.4) = Lu = b · u : @t x d Recall that a function f(x) is conserved if dt f(φt(x)) = 0. From (1:4) we learn that a function f is conserved if and only if (1.5) b · fx = 0: In the case of a Hamiltonian system, b = (Hp; −Hq) and the equation (1.5) becomes (1.6) ff; Hg := fq · Hp − fp · Hq = 0: 2 As an obvious choice, we may take f = H in (1.6). In general, we may have other conservation laws that are not so obvious to be found. Nother's principle tells us how to find a conservation law using a symmetry of the ODE (1.3). With the aid of the symmetries, we may reduce our system to a simpler one that happens to be another Hamiltonian-type system. Liouville discovered that for a Hamiltonian system of Nd-degrees of freedom (2Nd un- knowns) we only need Nd conserved functions in order to solve the system completely by means of quadratures. Such a system is called completely integrable and unfortunately hard to come by. Recently there has been a revival of the theory of completely integrable sys- tems because of several infinite dimensional examples (Korteweg{deVries equation, nonlinear Schr¨odingerequation, etc.). As we mentioned before, the conservation laws can be used to simplify a Hamiltonian system by reducing its size. To get more information about the solution trajectories, we may search for other conserved quantities. For example, imagine that we have a flow φt associated with (1:3) and we may wonder how the volume of φt(A) changes with time for a given measurable set A. For this, imagine that there exists a density function ρ(x; t) such that Z Z 0 (1.7) g(φt(x))ρ (x)dx = g(x)ρ(x; t)dx; for every bounded continuous function g. This is equivalent to saying that for every nice set A, Z Z (1.8) ρ(x; 0)dx = ρ(x; t)dx; φ−t(A) A 0 0 where ρ (x) = ρ(x; 0). In words, the ρ -weighted volume of φ−t(A) is given by the ρ(·; t)- weighted volume of A. Using (1.5), it is not hard to see that in fact ρ satisfies the (dual) Liouville's equation @ρ (1.9) + div(ρb) = 0: @t 0 As a result, the measure ρ (x)dx is invariant for the flow φt if and only if div(ρ0b) = 0: In particular, if div b = 0, then the Lebesgue measure is invariant. In the case of a Hamil- tonian system b = JrH, we do have div b = 0, and as a consequence, (1.10) vol(φt(A)) = vol(A); for every measurable set A. 3 In our search for other invariance properties, let us now look for vector fields F : Rn ! Rn such that d Z (1.11) F · dx ≡ 0; dt φt(γ) for every closed curve γ. Such an invariance property is of interest in (for example) fluid R mechanics because γ F · dx measures the circulation of the velocity field F around γ. To calculate the left-hand side of (1:11), observe Z Z F · dx = F (φt(x))Dφt(x) · dx φt(γ) γ where Dφt denotes the derivative of φt in x and we regard F as a row vector. Set F = 1 k 1 k 1 k (F ;:::;F ), φ = (φ ; : : : ; φ ), u = (u ; : : : ; u ), u(x; t) = TtF (x) = F ◦ φt(x)Dφt(x), so that X @φi uj(x; t) = F i(φ (x)) t (x): t @x i j To calculate the time derivative, we write u(x; t + h) = F (φt+h)Dφt+h = F (φt ◦ φh) Dφt ◦ φhD φh = u(φh(x); t)Dφh(x); so that i d j X i i @b u (x; t + h) = (ru · b)δij + u dh @x h=0 i j X @bi = ruj · b + ui @x i j X = (u · b) + (uj − ui )bi: xj xi xj i In summary, @u (1.12) = r(u · b) + C(u)b; @t where C(u) is the matrix [ui − uj ]. In particular, xj xi d Z Z (1.13) F · dx = C(u)b · dx; dt φt(γ) γ 4 for every closed curve γ. Recall that we would like to find vector fields F for which (1.11) is valid. For this it suffices to have C(F )b a gradient. Indeed if C(F )b is a gradient, then d Z d Z F · dx = F · dx dt φ (γ) dh φ (γ) t t+h h=0 Z d = F · dx dh φh(φt(γ)) h=0 Z = C(F )b · dx = 0: φt(γ) Let us examine this for some examples. Example 1.1. (i) Assume that k = 2n with x = (q; p) = (q1; : : : ; qn; p1; : : : ; pn). Let ∗ b(q; p) = (Hp; −Hq) = JrH for a Hamiltonian H(q; p). Choose F (q; p) = (p; 0). We then 0 I have C(F ) = n = J, and C(F )b = JJrH = −∇H. This and (1:13) imply that for −In 0 a Hamiltonian flow φt and closed γ, d Z (1:14) p · dq = 0; dt φt(γ) which was discovered by Poincar´eoriginally. ∗ (ii) Assume k = 2n + 1 with x = (q; p; t) and b(q; p; t) = (Hp; −Hq; 1) where H is now a time-dependent Hamiltonian function. Define F (q; p; t) = (p; 0; −H(q; p; t)): We then have 2 0 I H∗ 3 n q J (rH)∗ (1:15) C(F ) = −I 0 H∗ = : 4 n p 5 −∇H 0 −Hq −Hp 0 Since C(F )b = 0, we deduce that for any closed (q; p; t)-curve γ, d Z (1.14) (p · dq − H(q; p; t)dt) = 0; ds φs(γ) proving a result of Poincar´e and Cartan. Note that if γ has no t-component in (1:16), then (1:16) becomes (1:14). 5 2 3 0 −α3 α2 (iii) Assume n = 3. Then C(F ) = 4 α3 0 −α15 with (α1; α2; α3) = r × F . Now if −α2 α1 0 b = r × F , then C(F )b = (r × F ) × b and d R F · dx = 0. In words, the F -circulation dt φt(γ) of a curve moving with velocity field r × F is preserved with time. Example 1.2. A Hamiltonian system (1:2) simplifies if we can find a function w(q; t) such that p(t) = w(q(t); t). If such a function w exists, then q(t) solves dq (1:17) = H (q; w(q; t); t): dt p The equationp _ gives us the necessary condition for the function w: p_ = wqq_ + wt = wq · Hp(q; w; t) + wt; p_ = −Hq(q; w; t): Hence w(q; t) must solve, (1:18) wt + wq · Hp(q; w; t) + Hq(q; w; t) = 0: 1 2 For example, if H(q; p; t) = 2 jpj + V (q; t), then (1:18) becomes (1:19) wt + wqw + Vq(q; t) = 0: The equation (1:17) simplifies to dq (1:20) = w(q; t) dt in this case. If the flow of (1:20) is denoted by t, then φt(q; w(q; 0)) = ( t(q); w( t(q); t)). Now (1:14) means that for any closed q-curve η, d Z w(q; t) · dq = 0: dt t(η) This is the celebrated Kelvin's circulation theorem.
Details
-
File Typepdf
-
Upload Time-
-
Content LanguagesEnglish
-
Upload UserAnonymous/Not logged-in
-
File Pages181 Page
-
File Size-