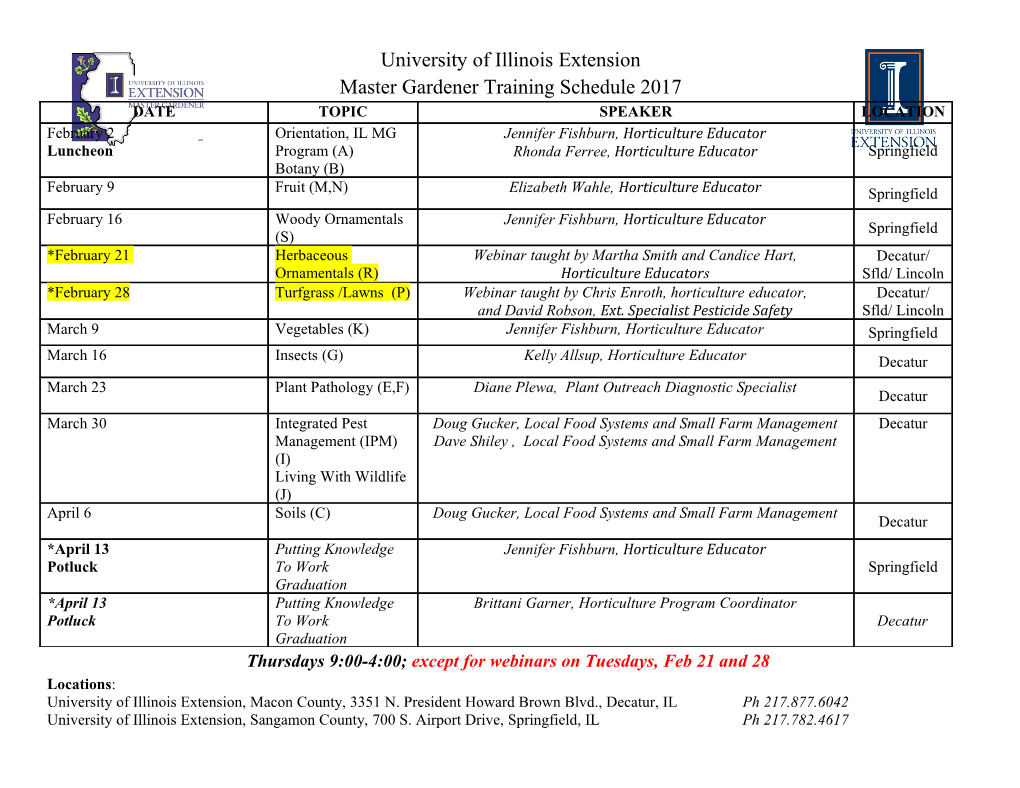
IMV1 on the Vortex Induced Vibrations of Flexible Flettner Rotors Literature Review Student- Michael Gillway Matriculation Number- S0929318 31/10/2013 1. Thesis Statement This project will investigate the conditions at which vortex induced instabilities are present on a flexibly supported, damped and rotating cylinder, which is allowed to pivot in the cross-flow direction and has a controlled stiffness. The intended outcome of these investigations will not only cover an area of research that has yet to be studied by experimental and computational means, but will also be of benefit to the fields of fluid mechanics and thrust generation. 2. Introduction Section 3 of this report will present a brief review into the background surrounding the Flettner rotor and its applications. A full account of the relevant literature surrounding this project will then be given in section 4; this will aim to establish a theoretical framework for the topic, defining the area of the study and identify the key findings in these areas. After doing so, the report will give an overview to a selection of preliminary design considerations and the progress report (up to the time of reporting). 3. Problem Formulation Flettner rotors are spinning cylinders, which produce fluid dynamic lift using the Magnus Effect. Anton Flettner- a German Aviation Engineer, used spinning cylinders for the first time as a ship’s propulsion system in 1926 (Lantham et al, 2012). In this voyage, the new and lighter rotors which replaced the previous sailing rig enabled the ship to break and turn around its own axis and to tack closer to the wind than its original design (Salter et al, 2008). However, with limitations in rotor bearing design (Salter et al, 2008), alongside the growth of the diesel engine and low fuel prices, the development of flettner rotor was stemmed. While these factors supressed the uses of the Flettner rotor in the early 20th century, the current market conditions, as well as the growing need for carbon dioxide mitigation has led to the appliance of Magnus technology across a range of sectors. This includes the Flettner ship itself, which has seen recent developments in a number of projects (Craft, 2013). An example of a current Flettner ship is the E-Ship 1, which was constructed in 2010 and is used by German wind turbine manufacturer Enercon GmbH (Enercon, 2010). The vessel employs four rotors which rise from the ships main deck and are used to power the ships propeller (Craft, 2013). Another poignant example is the proposed construction of a fleet of unmanned and flettner propelled vessels by Salter et al (2008). It is suggested that these vessels will utilise machine-cloud brightening technology to mitigate the effects of global warming. In addition to naval vessels, the magus effect has also been applied in the field of aeronautics. The rotor plane is a historic example and is a case where rotating cylinders were used in place of the traditional wing arrangement to provide the plane’s lifting force (Seifert, 2012). However, while designs such as the Plymoth A-A-2004 were tested as early as 1920 (Spooner, 1924), the power efficiency of the rotating cylinders were found to be lower than that of conventional wings (Seifert, 2012). Also, uncertainties into the structural strength of these systems meant the designs were never put into production (Seifert, 2012). Like the Flettner rotor, it was not until the late 20th century when the magus affect proved a useful solution for aeronautical applications. One of these is the third YOV-10A prototype proposed by Calderon (1961), which utilises Magnus technology on an aircraft to provide increased lift at low flying speeds. Further to the YOV-10A, rotating cylinders have also made their way into in the field of moving surface boundary layer control (MSBC) and have been implemented in aerofoil design concepts for flow control (Mittal, 2002). One example is the work of Modi (1990), who found that substantial increases in lift, delays in stall and suppression of the vortex-induced oscillations could be achieved by positioning rotating cylinders at the leading and/or trailing edge of the aerofoil. While it can be seen that rotating cylinders have a range of applications in thrust generation, Seifert’s review of the topic (Seifert 2012) concludes aeronautical applications to have numerous limitations and uncertainties into their operational stability and efficiency under a range of situations. The reporting into Rotor ships tells a different story. The E-Ship 1 is in current use and has been recounted to cover more than 17,000 sea miles through various waters and with no mention of instability (Enercon, 2013). Similarly, over the six years of operation, the functionality and reliability of the Flettner ship (named Babara) was proven and the rotors kept in good condition in adverse weather conditions (Seifert, 2012). Although these examples would support the stability of larger rotor ship designs, Barbara did encounter small amounts of ship heeling (less than 50) in adverse conditions (Seifert, 2012) and further to this, limited technical information can be accessed on the E-Ship 1. This topic was also addressed in a recent study by Latham et al (2012), who highlighted the need for examination into the effects of heeling on the aerodynamic performance of the Flettner rotor. The present work- identified in section 1- attempts to give a greater insight into the stability of rotating cylinders by experimental means and is expected to provide benefit to the engineering issues highlighted above. 4. Review of Literature Reid (1924) Prandtl (1925) and Thom (1926, 1932) were some of the first to measure the forces present on a spinning cylinder (Mittal, 2002). These experimental investigations were particularly concerned with the lifting characteristics of rotating cylinders and concluded that the mean lift of a rotating cylinder was a function of its rotation rate (Tokumaru et al, 1993). However, while informative, these initial efforts did not address how the flow characteristics changed as the cylinder speed was increased and the effect this had on the stability of the spinning system. In 1938, Goldstein (1938) published a study which showed that by increasing the circulation across a stationary circular cylinder, a flow pattern develops where fixed streamlines surround the cylinder. Following this discovery, a number of theoretical studies by Wood (1957), Moore (1957) and Glauert (1957) investigated the effect of circulation on rotating cylinders. These investigations were carried out for cases when the ratio of the surface speed of the cylinder to the free stream-speed of the fluid (termed α) was α=0. Wood, 1957, Moore, 1957, and Glauert 1957 all concluded that the rotation of the cylinder generated a circulation which was sufficient to delay flow separation for both finite and infinite (invicid) Reynolds flows. More so, the results of Wood, 1957, Moore, 1957, and Glauert (1957) showed that with a high enough value of α, it is possible supress vortex shedding across the cylinder. Within his initial research, Prandtl (1925) had proposed that the maximum circulation which could be realised about a rotating cylinder was equal to the circulation at which the upstream and downstream stagnation points joined (Tokumaru et al, 1993). From this analysis, he argued that the maximum value of the steady-state lift coefficient was a finite value of 4π (~12.6) in uniform flow. Prandtl’s proposal (1925) had obvious implications to applications using rotating cylinders for thrust generation. For this reason, a series of further investigations then aimed to examine the following two questions; if this was in fact the maximum value attainable by a rotating cylinder, and also, if the rotation of the cylinder can supress vortex shedding across range of α values. For cylinders rotating at α values between 0≤ α ≤ 1, Badr (1989), Dennis (1989) and Young (1989) confirmed that cylinder’s rotation could suppress flow separation, however, each study found differences in the lift and drag coefficients. In addition, Glauert (1957) also found that Prantl’s limit could be exceeded and argued that the circulation across the rotating cylinders increased indefinitely with α. Coutanceau and Menard (1985) experimentally investigated the problem of unsteady flows across rotating cylinders for Re=100, 500 and 1000 and 0.5≤ α ≤ 3.25. Past a critical α value (termed ) of approximately 2 and independent of Re, Coutanceau and Menard (1985) found that only one vortex was shed. This is supported by the computations from Badr and Dennis (1985) and the experimental results of Diaz et al (1983) who found that periodic vortices dissipate after a rotational rate of α=2 is reached. To investigate if the proposed by Coutanceau and Menard (1985) was true for higher Reynolds flows, an experimental and theoretical study was conducted by Badr et al (1990). This considered flows between 103<Re<104 and over a short time period of less than 22 seconds. Agreeing with the work of Coutanceau and Menard (1985), Badr et al (1990) found periodic vortex shedding to stop past a value of α=2. Further to this, Badr et al (1990) defined a steady region between the limits of 2<α<3, where vortex suppression was apparent. However, when a value of α=3 was reached, Badr et al (1990) found that three-dimensional flow effects resulted in vortex formation, and the experimental flow transitioned to turbulent. With the experiments of Badr et al (1990) showing vortex suppression due to α, Glauert’s (1957) proposal that circulation increases indefinitely with α, and the obvious advantages of higher lift generation, the question of Prandtl’s maximum lift was still of interest- as was the flow stability at higher α values.
Details
-
File Typepdf
-
Upload Time-
-
Content LanguagesEnglish
-
Upload UserAnonymous/Not logged-in
-
File Pages12 Page
-
File Size-