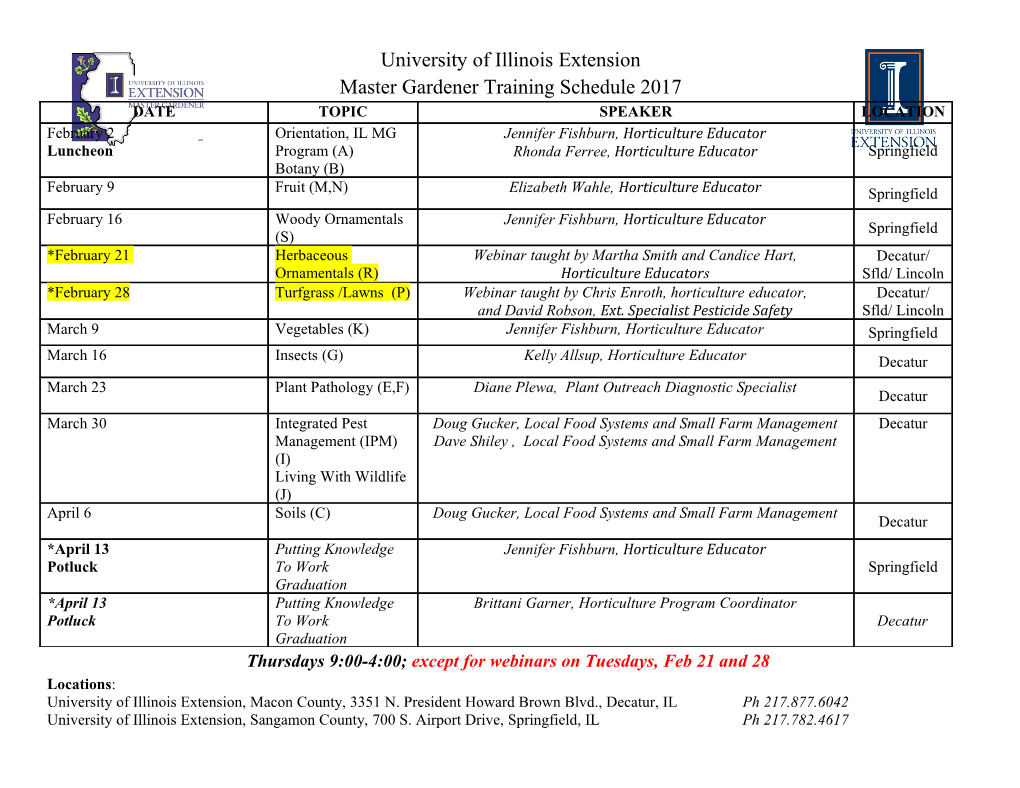
Quantum Information Science Raphael Pooser Oak Ridge National Laboratory Quantum Information Science Group and Quantum computing Institute A summary on quantum computing activities and their impact on the ORNL missions of scientific discovery and energy security. In collaboration with Travis Humble, Pavel Lougovski, Eugene Dumitrescu, Alex McCaskey, Ryan Bennink, Gaute Hagen, Thomas Papenbrock, Titus Morris ORNL is managed by UT-Battelle for the US Department of Energy Quantum Sensing and Quantum Computing Across Quantum Networks • Quantum networks are collections of qubits (nodes) connected by interactions, or quantum gates (edges) ∞ Sensing 퐸푃푅ۧ = න |푥ۧ푎 ⊗ |푥ۧ푏푑푥| • Simplest quantum network is the two qubit EPR state or −∞ Bell state, which is a workhorse in quantum sensing Concatenated EPR states • The quantum correlations in EPR quantum networks can be used to reduce the noise floor in measurements – quantum metrology Classical noise limit … Lead to square cluster states Signal obscured by classical limit Quantum … … noise limit … Which can be tiled • Indefinitely large quantum networks can be built by Measurement and feedforward Computing concatenating EPR states – the same network is a enacts quantum resource for measurement-based quantum computing gates And informs subsequent and distributed quantum sensors measurements The know-how in generating long range entanglement for quantum sensing lends itself to building quantum computers. This is because in order to make these quantum sensors, one must build a quantum network with a two qubit gate interaction between the nodes. Quantum Computing at ORNL • Scientific discovery and energy security with quantum computing Physical Sciences: Chemistry, Applied Sciences: Energy, Data Sciences: Applied Math, Materials Sciences, Physics Medicine, Engineering Analytics, Artificial Intelligence, Electronic structure calculations of Finite-element methods for multi- Machine learning methods will nitrogenase will support methods for scale models will support integrated support data analytics and artificial fertilizer production, food security computational materials engineering intelligence for situational awareness 3 Quantum Computing Institute Quantum Computational Science • Quantum computing provides a step change in capability for scientific discovery and energy security Algorithms in the quantum computing model have been found to take significantly fewer steps to solve key problems • Quantum Simulation • Factoring • Partition Functions • Unstructured Search • Discrete Optimization • Eigensystems • Machine Learning • Linear Systems Multiple application domains motivate quantum computing as a paradigm for scientific computing • High-energy Physics • Artificial Intelligence • Materials Science • Data Analytics • Chemistry • Planning and Routing • Biological Systems • Verification and Validation 4 Quantum Computing Institute The Impact on Energy Security Haber-Bosch Process • Fertilizer production consumes ~5% of global natural gas supplies and 2% of global energy production – This is necessary to meet food requirements worldwide – The artificial Haber-Bosch process requires extremely high heat and pressure to achieve efficient ammonia production • The nitrogenase enzyme operates at STP and is used by bacteria for ammonia production + − N2 + 8 H + 8 e → 2 NH3 + H2 • Quantum computing will enable high fidelity modeling of these complex chemical reactions – Quantum algorithms for computational chemistry provide exponential speed ups over conventional approache 5 Quantum Computing Institute Quantum Computing LDRD Investments • We are building new capabilities for quantum materials, devices and systems through strategic investments (FY16-18) Materials Devices Systems P donor in Si (Humble, Lupini) Qubit Compiler (McCaskey) Qubit operations Graphene Qubit (Peters) (Jesse) Model, M 77 K 77 K 4.2K quantum 4.2K 100 K interconnect 100 K stage stage classical control control Quantum/Classical Sub-radiant Interfaces Prediction Data, Dt Classical End User (Lougovski) Dissipative QC 300 K Super-radiant (Evans) Dilution Dilution Qubit fidelity modeling Fridge A Fridge B 6 Quantum Computing Institute (Bennink) Cluster-State Quantum Computing Measurement-based quantum computing relies on a large resource called a cluster state, which consists of a large number of entangled modes. Measurements are used to propagate both single and two-qubit gates, resulting in a very simple implementation. Continuous-variable implementations allow for deterministic state preparation. • Optical, continuous variable cluster states have demonstrated 1,000,000 entangled nodes • To date no implementations have allowed all nodes to be individually addressable, and complex experiments requiring multiple phase locks mean that very few demonstrations have ever been made • Our implementation requires no lock loops and allows all generated nodes to be individually addressable without multiplexing, paving the way to truly scalable QC. Supported by ORNL LDRD program. Dual rail cluster state Four node entangled cluster state … 7 Quantum Computing Institute Pooser, Jing, Physical Review A 90, 043841 (2014) Lawrie et al., Applied Physics Letters 108, 151107 (2016) ASCR Early Career Award in Quantum Computing • We are developing long-term capabilities in the Shared Memory Machine design and operation of quantum computing system within DOE HPC facilities. QPU • The goal of the project is to accelerate existing scientific applications using quantum Interconnect processors. The approach relies on physics, Node Node Node engineering, and computer science to QPU QPU QPU demonstrate quantum-classical programming and run-time environments for scientific Interconnect applications. Node Node Node • ORNL PI Travis Humble QPU QPU QPU • DOE PM Robinson Pino Quantum Interconnect 8 Quantum Computing Institute ASCR Quantum Algorithms Team (FY17-FY20) • We are developing near-term applications of quantum computing for solving scientific problems including quantum many-body systems in superconducting materials, lattice QCD, and nuclear physics. • The team of ORNL staff and subject matter experts from University of Washington and University of Bilbao focuses on demonstrating quantum computation and improving performance through algorithmic design. • ORNL PI Pavel Lougovski • DOE PM Ceren Susut 9 Quantum Computing Institute ASCR Quantum Testbed Pathfinder (FY17-FY22) • We are exploring the design and development of quantum computing for scientific applications in chemistry, nuclear physics, and machine learning. • The team consists of ORNL staff and a consortium of quantum computing stakeholders, including IBM, Google, and IonQ, focused on demonstrating and characterizing application development. • ORNL PI Raphael Pooser • DOE PM Claire Cramer 10 Quantum Computing Institute Quantum Computing Testbed Pathfinder • Currently two performers: LBNL and ORNL • Our project: Methods and Interfaces for Quantum Acceleration of Scientific Applications • We will determine the feasibility of running scientific applications of interest to ASCR and DOE on near-term quantum computer hardware. One of our jobs is to foster industry relationships and gain access to multiple quantum computers as they come online, harnessing them for DOE’s purposes. • Key partners: IBM Q, ionQ, GaTech, VaTech • ORNL’s long term goal is to host a quantum computer testbed on site. Our relationship with industry partners will bootstrap the process. 11 Quantum Computing Institute Quantum Computing User Community • We are providing state-of-the-art quantum computing resources to the user community through strategic partnerships, collaborations, and on-site resources Universities Industry Government 12 Quantum Computing Institute Architectures What we mean by architecture: We will study the make-up of a quantum computer in terms of its fundamental gate set at the physical level and higher levels of abstraction, in terms of its interconnectivity amongst the qubits present on the chip, and in terms of the actual physical qubit substructure. 13 Quantum Computing Institute Architectures: superconductors and ion traps 14 Quantum Computing Institute Architectures: superconductors and ion traps But: maintain open mind and bring other qubits in as they come online; partner with industry leaders in qubit technology! 15 Quantum Computing Institute Feasibility What we mean by feasibility: We will study an array of performance metrics associated with each application, and these metrics will be correlated with the characteristics of the hardware architectures. To achieve high VQ one must achieve equal depth and width. No credit is given for circuits deeper than n. 16 Quantum Computing Institute 17 Presentation name Codesign • Architecture dictates the overlap with desired application space • Hardware is capable of certain low level operations which can be strung together to create algorithms, which are strung together to make an application • Feedback from application (software) performance to hardware design can expose new capabilities 18 Quantum Computing Institute Interfaces • Various levels of testbed stack supported by DOE/ORNL projects 19 Presentation name Interfaces Algorithm instantiation • High level language (e.g. Quipper) • Compilation to QASM • New algorithm development • Never-before-seen algorithms • Variational ansatz development QATs program 20 Presentation name Interfaces Testbed program At the interface between hardware and software: • Control signals (e.g., waveforms) • Low level API
Details
-
File Typepdf
-
Upload Time-
-
Content LanguagesEnglish
-
Upload UserAnonymous/Not logged-in
-
File Pages38 Page
-
File Size-