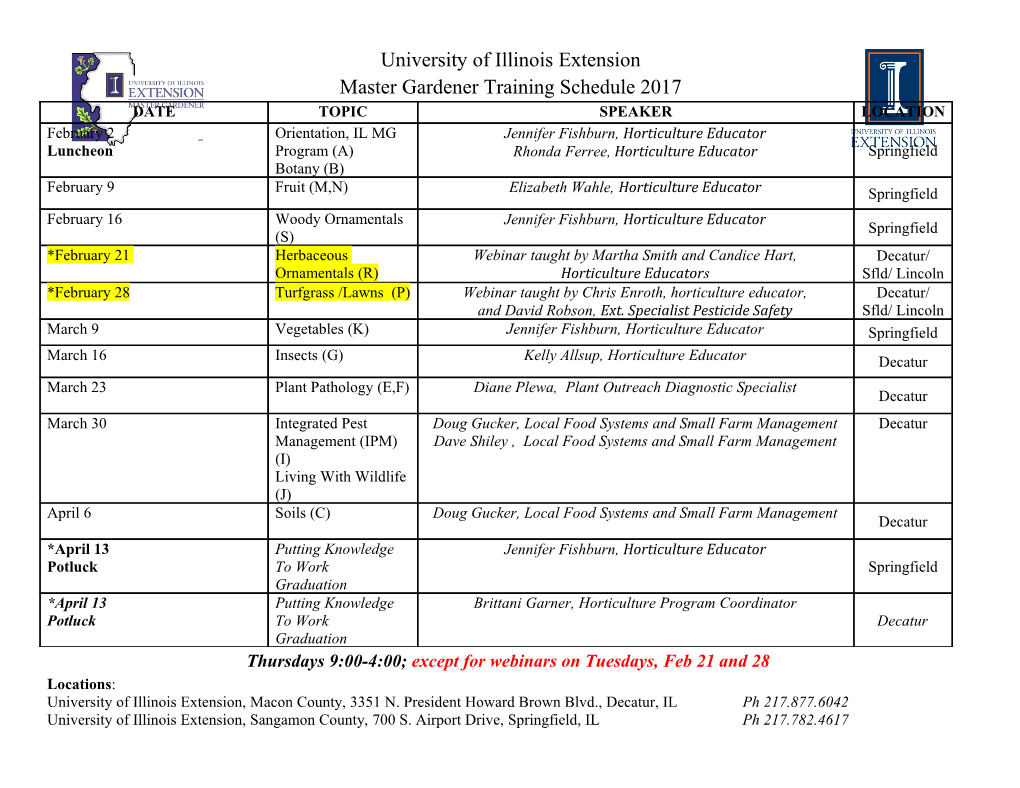
Evolution of the coeloconic sensilla in the peripheral olfactory system of Drosophila mojavensis A thesis submitted to the Graduate School of the University of Cincinnati in partial fulfillment of the requirements for the degree of Master of Science in the Department of Biological Sciences of the College of Arts and Sciences by Daniel Carl Nemeth B.S. in Zoology Miami University May 2015 Committee Chair: S. M. Rollmann, Ph.D. i ABSTRACT Distinct environmental conditions characterized by habitat, climate, resource availability, predation, and competition can result in the development of adaptive traits through natural selection. Divergence of adaptive traits can be seen in the olfactory systems of phytophagous insects with differing host plant preferences among conspecific populations. In this study, we examine the cactophilic fly, Drosophila mojavensis, which utilizes different host cacti across its range. Each cactus emits its own, unique composition of volatiles, which may serve as a primary sensory cue for host plant localization. Specifically, we study the olfactory system of these flies in attempts to elucidate mechanisms underlying the reported phenotypic divergence among populations. We observed variation in odor-evoked electrophysiological responses of acid and amine sensing olfactory sensory neurons (OSNs) of coeloconic sensilla across all sensillar types among populations. Additionally, we observed loss of expression an odorant receptor gene, Or35a in all four populations. Expansion to other Drosophila species revealed comparable absences in OSN responses to OR35a agonists in D. virilis and D. arizonae, suggesting a potential similar loss in gene expression as seen in D. mojavensis. Furthermore, responses of ac3 OSNs significantly differed to sixteen of the seventeen odorants tested across the four drosophilid species. Collectively, our results highlight evolution of acid and amine sensing in coeloconic sensilla through peripheral changes observed in Drosophila olfactory systems. ii © 2017 Daniel Carl Nemeth All rights reserved iii ACKNOWLEDGMENTS The fruition of this thesis would have not been possible without the help and support of so many great people. First and foremost, I need to thank my brilliant advisor, Dr. Stephanie M. Rollmann, who not only supported me as an educating mentor, but pushed and motivated me to accomplish much more than I thought I was capable of. The skills I have learned during my graduate career under her guidance, will be carried with me onto my future endeavors. I would also like to thank my two committee members, Dr. John E. Layne, for his expertise on electrophysiology and all his help with the statistical analysis of my project, and Dr. Ann M. Ray, for her insightful critiques and assistance as we determined the full construct of my research project. I would like to extend a thanks to all of my lab colleagues during my graduate work at the University of Cincinnati, especially my lab mates Dr. Elizabeth Brown, Amber Crowley-Gall, Vishnu Gomez, and Cody Patterson for their helpful discussions about my research, as well as their help with any fly work if I was out of town, as to not delay my data collection upon returning. Also, a special thanks to Dr. Byrappa Ammagarahalli Munishamappa, for his electrophysiological knowledge and help with the categorization of ac4 sensilla, as well as his company during the countless of hours of our antennal dissections. Lastly, I would like to extend many thanks to my parents, Meg and Bill, my siblings and in-laws, Jen, Greg, Chris, April, and Steph as well as my relatives and close friends for their constant, loving support and encouragement throughout my graduate career and my life. I would not be where, nor who I am today without their help, and I cannot thank them enough. iv TABLE OF CONTENTS ABSTRACT ............................................................................................................................. ii ACKNOWLEDGMENTS ..................................................................................................... iv TABLE OF CONTENTS ....................................................................................................... v LIST OF TABLES & FIGURES .......................................................................................... vi 1. INTRODUCTION ............................................................................................................... 1 2. MATERIALS & METHODS ........................................................................................... 11 2.1 Fly stocks ....................................................................................................................... 11 2.2 Electrophysiology .......................................................................................................... 11 2.2.1 Odorant stimuli ....................................................................................................... 11 2.2.2 Single sensillum recordings (SSR) .......................................................................... 12 2.3 Gene expression analysis: RT-PCR ............................................................................... 13 2.4 DNA sequencing of ionotropic receptor coding regions ............................................... 14 3. RESULTS .......................................................................................................................... 15 3.1 Characterization of OSN response profiles for coeloconic sensilla............................... 15 3.2 Electrophysiological differences among D. mojavensis populations ............................ 16 3.3 Olfactory receptor gene expression of predicted ac3 sensilla orthologs........................ 17 3.4 Population differences in olfactory receptor gene sequence ......................................... 18 3.5 Species differences in electrophysiological responses of the ac3 sensilla ..................... 18 4. DISCUSSION .................................................................................................................... 20 5. REFERENCES .................................................................................................................. 29 6. FIGURE LEGENDS ......................................................................................................... 40 v LIST OF TABLES & FIGURES Table 1. List of chemical compounds used in electrophysiological recordings ............... 42 Table 2. RT-PCR Primers. ................................................................................................ 43 Figure 1. Single sensillum recordings of D. mojavensis populations .............................. 44 Figure 2. OSN classification with known D. melanogaster odor response spectra. ........ 45 Figure 3. Statistical differences in electrophysiological responses among the four D. mojavensis populations. .................................................................................................... 46 Figure 4. Single sensillum recording from an ac3 sensillum. .......................................... 47 Figure 5. RT-PCR analysis of ionotropic receptor gene expression in all four D. mojavensis populations. .................................................................................................... 48 Figure 6. (a) Amino acid sequence differences in IR75a among D. mojavensis populations. ....................................................................................................................... 49 Figure 6. (b) Amino acid sequence differences in IR75bc_GI13611 among D. mojavensis populations .........................................................................................................................50 Figure 7. (a, b) Single sensillum recordings of drosophilid species. ............................... 51 vi 1. INTRODUCTION The process of environmental adaptation is a driving force in evolution, which encompasses the ability of an organism to survive in differing environments. Organisms that have colonized new environments may acquire specific adaptive traits that better suit them to local conditions. Differences in environmental conditions such as habitats (terrestrial and aquatic), climate (temperature and precipitation), resource availability (food and breeding sites), predation, and competition, can apply differing selection pressures, which may differentially adapt populations to different habitats (Schluter, 2001; Linn et al., 2003; Rundle and Nosil, 2005). This process may lead to morphological, physiological, and behavioral shifts, and divergence in traits between populations in natural conditions (Funk, 1998; Schluter, 2001; Fuse, 2017). These shifts have been documented in the lab through artificial environmental adaptation as well. For instance, a genetic line of the fly Drosophila melanogaster, dubbed ‘Dark fly’ because it has been reared in the dark for over 1500 generations, exhibited divergent morphological, physiological, and behavioral characteristics in its sensory system in response to living in complete darkness, aiding its ability to thrive under these environmental conditions (Fuse et al., 2014; Fuse, 2017). With the genetic resources available for D. melanogaster, ‘Dark fly’ not only presents a valuable genetic tool in our quest to better understanding the mechanisms underlying environmental adaptation in nature, but simply demonstrates the ability of how organisms are able to adapt. Differential adaptation in response to distinct environmental conditions between
Details
-
File Typepdf
-
Upload Time-
-
Content LanguagesEnglish
-
Upload UserAnonymous/Not logged-in
-
File Pages58 Page
-
File Size-