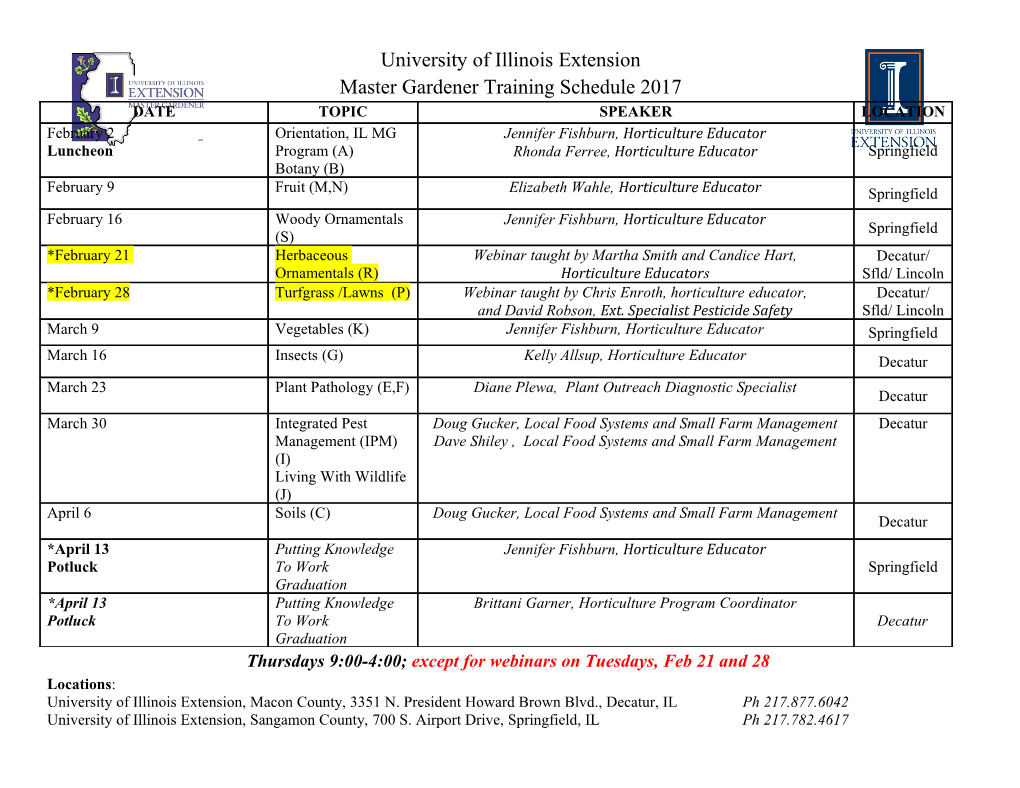
J Physiol 579.3 (2007) pp 613–619 613 SYMPOSIUM REPORT Brain–computer interfaces as new brain output pathways Jonathan R. Wolpaw Wadsworth Center, Laboratory of Nervous System Disorders, New York State Department of Health and State University of New York, Albany, NY 12201, USA Brain–computer interfaces (BCIs) can provide non-muscular communication and control for people with severe motor disabilities. Current BCIs use a variety of invasive and non-invasive methods to record brain signals and a variety of signal processing methods. Whatever the recording and processing methods used, BCI performance (e.g. the ability of a BCI to control movement of a computer cursor) is highly variable and, by the standards applied to neuromuscularcontrol,couldbedescribedasataxic.Inanefforttounderstandthisimperfection, this paper discusses the relevance of two principles that underlie the brain’s normal motor outputs. The first principle is that motor outputs are normally produced by the combined activity of many CNS areas, from the cortex to the spinal cord. Together, these areas produce appropriate control of the spinal motoneurons that activate muscles. The second principle is that the acquisition and life-long preservation of motor skills depends on continual adaptive plasticity throughout the CNS. This plasticity optimizes the control of spinal motoneurons. In the light of these two principles, a BCI may be viewed as a system that changes the outcome of CNS activity from control of spinal motoneurons to, instead, control of the cortical (or other) area whose signals are used by the BCI to determine the user’s intent. In essence, a BCI attempts to assign to cortical neurons the role normally performed by spinal motoneurons. Thus, a BCI requires that the many CNS areas involved in producing normal motor actions change their roles so as to optimize the control of cortical neurons rather than spinal motoneurons. The disconcerting variability of BCI performance may stem in large part from the challenge presented by the need for this unnatural adaptation. This difficulty might be reduced, and BCI development might thereby benefit, by adopting a ‘goal-selection’ rather than a ‘process- control’ strategy. In ‘process control’,a BCI manages all the intricate high-speed interactions involved in movement. In ‘goal selection’,by contrast, the BCI simply communicates the user’s goal to software that handles the high–speed interactions needed to achieve the goal. Not only is ‘goal selection’ less demanding, but also, by delegating lower-level aspects of motor control to another structure (rather than requiring that the cortex do everything), it more closely resembles the distributed operation characteristic of normal motor control. (Received 1 December 2006; accepted after revision 10 January 2007; first published online 25 January 2007) Corresponding author J. R. Wolpaw: Wadsworth Center, New York State Dept. Health, PO Box 509, Empire State Plaza, Albany, NY 12201-0509, USA. Email: [email protected] Introduction muscular dystrophies, and cerebral palsy (Wolpaw et al. 2002; Wolpaw & Birbaumer, 2006 for review). All other Brain–computer interfaces (BCIs) are a fundamentally assistivetechnologymethodsdependonthebrain’snatural new approach to restoring communication and control to output pathways of peripheral nerves and muscles (or people with severe motor disorders such as amyotrophic peripheral nerves and glands; Wilhelm et al. 2006), and lateral sclerosis (ALS), brainstem stroke, spinal cord injury, take outputs that the person still retains (e.g. vertical eye movement in a person with a brainstem stroke) and use This report was presented at The Journal of Physiology Symposium these to replace missing functions (e.g. using gaze direction on Physiology of brain–computer interfaces, Atlanta, Georgia, USA, 13 to select letters on a computer screen). In contrast, BCIs October 2006. It was commissioned by the Editorial Board and reflects give the brain entirely new output pathways. They take the views of the author. electrophysiological or other measures of brain activity C 2007 The Author. Journal compilation C 2007 The Physiological Society DOI: 10.1113/jphysiol.2006.125948 614 J. R. Wolpaw J Physiol 579.3 and from these measures determine the person’s wishes. difficulty and how it might be at least circumvented and at Intent, which is normally achieved by speaking or by best overcome are the topic of this article. another motor action, is instead achieved by producing brain signals that encode the intent so that a computer can translate it into control of a device such as a computer cursor or a neuroprosthesis. Limitations of current BCIs BCI research, which was confined to only three groups BCI studies usually take place in highly controlled 20 years ago and only six to eight groups as recently as laboratory environments or in similarly constrained 10 years ago, is now a burgeoning enterprise, with over clinical situations. The BCI user, whether human or 100 groups throughout the world engaged in a broad animal, typically assumes a specific posture in a simple spectrum of research and development efforts, and more stereotyped setting free of distractions and operates the entering the field every month. Up to now, this work BCI for brief periods under close supervision. In spite has demonstrated that a variety of different brain signals, of these controlled conditions, one of the hallmarks of recorded in a variety of different ways and analysed with the results achieved is their variability. Users do much a variety of different algorithms, can support some degree better on some days than others, and performance can of real-time communication and control (Vaughan & vary widely even within a single session and from trial Wolpaw, 2006). As a result of this collective effort, two to trial. This high variability is perhaps best illustrated facts are becoming increasingly clear. One is encouraging, by BCI-based movement control. For example, Fig. 1 the other is sobering. compares cursor movement times when the cursor is First, BCIs do offer a potentially valuable new option controlled by a joystick to cursor movement times when for restoring communication and control to people with the cursor is controlled by a set of single neurons in severe disabilities; and practical dissemination of BCI motor cortex (Hochberg et al. 2006). BCI control is slower technology has in fact begun. Second, however, the than joystick control and is also far more variable. Such development of BCIs that are at once practical, reliable variability appears to be a characteristic feature of all BCI and capable of high-speed complex communication and approaches, whether non-invasive (e.g. EEG) or invasive control is an enormously difficult problem, and one that is (e.g. electrocorticographic (ECoG) or intracortical). In far from solution. Furthermore, the origin of the difficulty spite of prolonged practice and frequent recalibration is not clear – it is not simply a need for better recording of the algorithms that translate brain signals into methods or improved analysis algorithms – and thus the output commands, variability in performance remains best route to its solution is also not clear. The origin of this substantial. In contrast to the actions carried out by the brain’s normal neuromuscular pathways, which are very consistent from trial to trial (e.g. Fig. 1), the actions carried 140 out through BCIs display a disconcerting, and, up to now 120 at least, ineradicable variability. This variability is likely to be even greater when BCIs are taken out of the protected 100 settings in which they are now typically used and are 80 applied to the day-to-day needs of people with severe disabilities. Count 60 Studies of multidimensional movement control are 40 revealing another surprising feature of BCI performance. Although most researchers had assumed, and many still 20 assume, that invasive methods that use single-neuron activity will provide far better control than non-invasive 0 01234567methods that use EEG, the results to date do not Time (Seconds) support this assumption. As Table 1 summarizes, the movement control obtained with scalp-recorded Figure 1. Distributions of times needed to move the cursor to the target in a centre-out task for a person using neurons in sensorimotor rhythms falls in the same range in motor cortex (dashed line) and for three people using a joystick terms of speed and precision as the control obtained (continuous line) with single neurons. The similarity is strikingly The arrows indicate the median times for each distribution. The neuron illustrated by comparing the videos available at http:// control data are from 6 daily sessions, and include only the 73–95% www.bciresearch.org/html/2d control 8tn.html (Wolpaw (depending on the session) of the trials in which the cursor reached the target within 7 s. Even when the trials taking > 7 s are ignored, neural & McFarland, 2004) and http://www.nature.com/nature/ control is substantially slower and much more variable than normal journal/v442/n7099/suppinfo/nature04970.html (video muscle control. (From Hochberg et al. 2006; reprinted by permission 1) (Hochberg et al. 2006). Both show centre-out cursor from Macmillan Publishers Ltd: Nature vol. 442, pp. 164–171, C 2006.) control, one with EEG and one with single neurons. C 2007 The Author. Journal compilation C 2007 The Physiological Society J Physiol 579.3 BCIs as new brain outputs 615 Table 1. Comparison of BCI point-to-point movement control achieved with intracortical micro- electrodes that record single neuron activity (Serruya et al. 2002; Taylor et al. 2002; Carmena et al. 2003) and EEG scalp electrodes that record sensorimotor rhythms (Wolpaw & McFarland, 2004) Movement Movement Hit Method Reference time precision rate (s) (%) (%) Intracortical Serruya et al. (2002) 1.5–2.2 1.3–7.7 86–89 implant Taylor et al. (2002) Carmena et al. (2003) Scalp EEG Wolpaw & McFarland (2004) 1.9 4.9 92 The ranges are based on each study’s best user.
Details
-
File Typepdf
-
Upload Time-
-
Content LanguagesEnglish
-
Upload UserAnonymous/Not logged-in
-
File Pages7 Page
-
File Size-