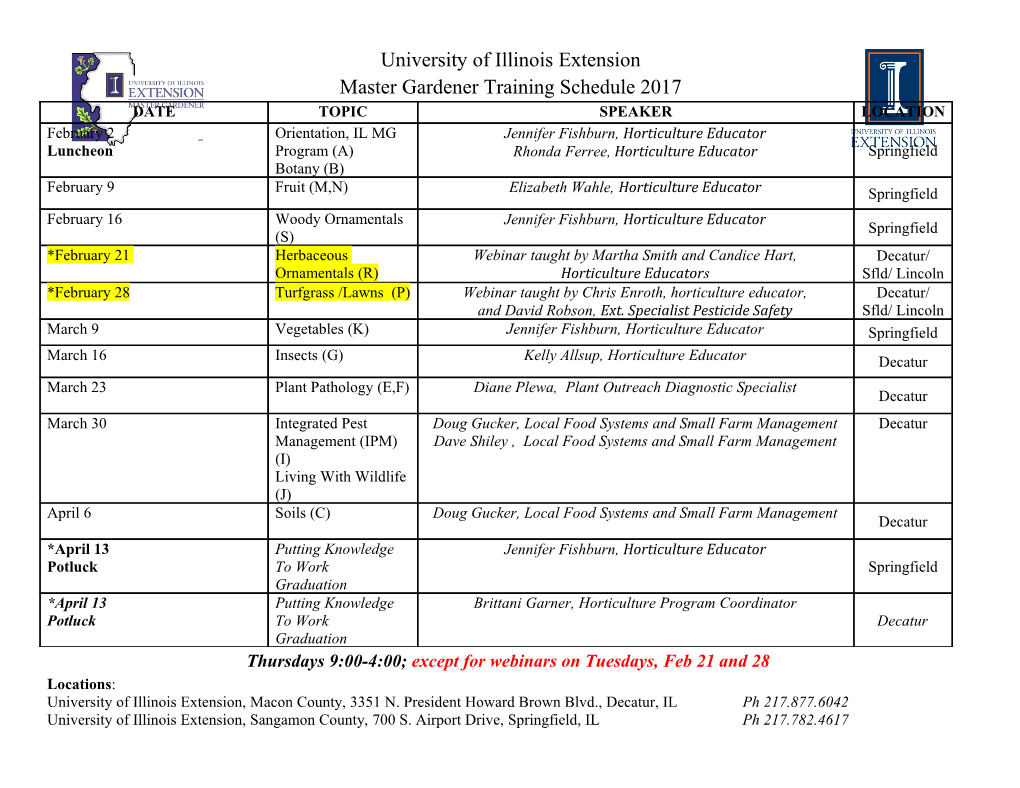
6 Carbon and Hydrogen Isotopic Effects in Microbial Methane from Terrestrial Environments Jeffrey Chanton, Lia Chaser, Paul Glasser, Don Siegel Methane is the ultimate end-product of anaerobic respiration of organic matter. In terrestrial freshwater systems it is formed by two main pathways, fermentation of acetate CH3COOH --+ CH4 4- CO2 (6.1) and reduction of CO2 with hydrogen 2CH20-4- 2H20 --+ 2CO2 -4-4H2 (6.2) CO2 4- 4H2 --+ CH4 4- 2H20 (6.3) which results in Eq. 6.4, overall. 2CH20 --+ CH4 4- CO2 (6.4) Note that CO 2 reduction results in overall net CO2 production when the production of hydrogen is accounted for. Methane production via CO2 reduction does not consume CO2. Also, acetate can be written as 2CH20, so Eq. 6.4 describes both pathways. Acetate, H 2 and CO2 are generated in organic-rich environments by the respiration of anaerobic and fermentative bacteria higher in the anaerobic food web. Methane is the perfect waste product: it is non-toxic and relatively insoluble in water so it forms bubbles and is rapidly removed via ebullition (Martens and Klump, 1980) or transported via vegetation (Dacey, 1981; Chanton and Dacey, 1991). When CH4 migrates to an oxygen interface (Brune et al., 2000), it is rapidly consumed by methano- trophic bacteria (King, 1992) and can support chemosynthetic communities (Paull et al., 1989; Martens et al., 1991). An important functional relationship of low temperature geochemistry is that there are coincidental shifts in the 613C and 6D of methane isotopic composition relative to the methane production pathway and/or to the 85 86 6. Carbon and Hydrogen Isotopic Effects CO 2 -200- reduction . -400 - Acetate fermentation I I -80 -60 ~130 (%o) Figure 6.1 Scheme of variations in al3c and aD associated with methane oxidation and production mechanisms. The lower right corner of the graph represents acetate fermenta- tion while the upper left corner represents CO 2 reduction. Oxidation effects leave residual methane enriched in 13C and D isotopes. effects of microbially mediated methane oxidation (Fig. 6.1). The purpose of this chapter is to illustrate these shifts as they occur in terrestrial environ- ments and to consider the factors that influence this relationship, including anthropogenic impacts (e.g., landfills). As seen in Fig. 6.1, changes in 13CH4 alone are ambiguous, forced either by methane oxidation (Barker and Fritz, 1981) or variation in the methane production mechanism (Sugimoto and Wada, 1993). Information on variation in aD of CH4 should strengthen any interpretation of 13C data (see for example Woltemate et al., 1984; Whiticar and Faber, 1986; Martens etal., 1992; Kelley etal., 1995; Bellisario etal., 1999). While C and H isotope systematics can be useful for diagnosing a wide variety of processes leading to methane production (Whiticar et al., 1986; Whiticar, 1993, 1999), this chapter will focus only on microbial methane produced or consumed in low temperature settings. Knowledge of the isotopic composition of source methane emitted from natural and anthropogenic systems is helpful for developing a global budget for methane sources and sinks (Fig. 6.2) (Stevens and Engelkemeir, 1988; Wahlen et al., 1989; Tyler, 1991). As stated by Miller (2004), a better under- standing of the processes responsible for determining isotopic fractionation during both production and consumption of methane will allow better constraints on our estimates of the sources and sinks in the global methane budget. Isotopic Effects During Methane Oxidation When 13C and D isotopes of CH4 shift in the same direction, for example when methane becomes more enriched in both 1~C and D along a spatial or temporal gradient, the variation is referred to as sympathetic. Sympathetic Isotopic Effects During Methane Oxidation 87 Rice Tropical wetlands Sewage and liquid nimals Fens waste Combustion and Bogs Coal biomassburning n , ,1 I I I I -70%o -20% Amazon floating Gas venting meadows -53.2%o Figure 6.2 A representation of the mass-weighted isotope mass balance approach for atmospheric methane inputs (redrawn from Chanton et al., 2000). The representation is similar to a 'see-saw' in that both the size of the bar and distance from the fulcrum point influence the importance of the source. The x-axis (the solid line resting on the fulcrum) represents the ~13C of the methane source, and the total size of the bars is roughly related to the source strength in this representation. Bar thickness represents the S13C range of the source. The position of the fulcrum represents the isotopic composition of the global methane input, which is determined from the values of atmospheric methane corrected for isotopic fractionation associated with removal processes. The sizes of the bars in this representation are for illustrative purposes for the most part and only roughly depict the mass of the fluxes. variations are typically considered to indicate the activity of methane- oxidizing bacteria, methanotrophs (Fig. 6.3). These bacteria preferentially consume CH4 containing the lighter isotopes, leaving residual CH4 that is enriched in the heavier isotopes, 13C and D. The fraction of CH4 that is oxid- ized can be calculated from changes in CH4 isotopic composition across a spatial gradient from anoxic to oxidizing conditions (Happell et al., 1994; Liptay et al., 1998; Chanton et al., 1999; Chanton and Liptay, 2000). Sympathetic shifts in the 13C and D isotopic composition of methane associated with oxidation were first reported by Coleman et al. (1981) in incubation studies, and subsequently have been verified in a number of field studies. The amount of the D shift is always greater than for the C shift, because the mass change of 1 is proportionally greater for H than it is for C. This means that the fractionation factor, ~H for hydrogen-deuterium is larger than the fractionation factor for carbon, ~C. Isotopic fractionation in this case is a kinetic process so the term ~ is defined as 0t = kL/kH (6.5) where kL refers to the first-order rate constants for the reaction of 12CH4 or CH4, and kH refers to the rate constants of 13CH4 and CH3D. When c~ is expressed in this manner, it is greater than 1 since the molecules containing the lighter isotopes react faster than those containing the enriched or heavier elements; estimates of ~C for aerobic methane oxidation vary from 1.008 to 1.031 (Reeburgh, 1996; Liptay et aL, 1998; Chanton and Liptay, 2000). 88 6. Carbon and Hydrogen Isotopic Effects -175 [] - 200 [] / -225 -250 1211-1 -275 [] -325 ', , , , , , , -65 - 55 -45 - 35 -25 •130 %0 Figure 6.3 Effect of methane oxidation on 613C and 6D of CH 4. Samples from several landfills in New England (Liptay et aL, 1998). Filled circles represent anoxic zone methane which has not been subject to oxidation. Chamber samples (open squares) captured emitted methane which has experienced the activity of methane-oxidizing bacteria. The slope of the line fit to the data is 3.8. Some measurements of the size of the A~D/A~13C shift for CH4 oxidation are tabulated in Table 6.1. Interestingly, observations from the field are smaller than initial laboratory results. Further investigation comparing 61~C and 3D fractionation driven by methanotrophy in lab and field studies is needed. Isotopic Effects Associated with Methane Production Mechanisms Variation in the two predominant mechanisms of methane production, CO2 reduction and acetate fermentation, has been hypothesized to be associated with inverse or antipathetic shifts in 6D and al~c of CH4 (Fig. 6.1; Table 6.1 Examples of Relative Changes in ~D of CH4 Relative to 13C of CH 4 Caused by Methanotrophic Activity Environment AaD/A813C References Landfill soil 2.5-3.7 Liptay et al. (1998) Landfill soil 4 Lubina et al. (1996a,b) Laboratory incubations 8.5-13.5 Coleman et al. (1981 ) Wetlands 5.5 Happell et al. (1994) Wetlands 2.5 Burke et al. (1988b) Isotopic Effects Associated with Methane Production Mechanisms 89 Whiticar et at, 1986; Schoell, 1988; Burke et at, 1988a,b; Whiticar, 1993, 1999; Hornibrook et al., 1997). Carbon isotope separation between CO2 and CH4 ranges from 60 to 90%0 for CO2 reduction and 40 to 60%0 for acetate fermentation (Whiticar, 1999; Conrad et al., 2002). Hydrogen isotope effects lead to CH4-H20 separation of 170 to 250%0 for CO2 reduction and 250 to 400%0 for acetate fermentation. The hypothesis is that if all things are relatively equal, fermentation of acetate will result in CH4 which is 13C- enriched and D-depleted relative to CH4 produced via the CO2 reduction pathway. There are two key words in the pronouncement above, 'relatively equal.' Above we referred to understanding the changes in CH4 isotopic com- position along a gradient. The scale of this gradient must be sufficiently small that the isotopic composition of methane precursors does not change significantly in such a way that interpretation will be compromised. For example, Waldron et al. (1999) demonstrated that on a grand spatial gradi- ent from the tropics to the poles, methane aD composition is controlled by the aD of water present. However, at smaller spatial scales, where the aD of water is fairly constant, aD variation of CH4 is most likely due to variation in the oxidation or production mechanism, as discussed below. Similarly, the 613C of methane can be affected by the isotopic composition of the parent organic matter. Methane produced from the decay of C3 vegetation has been observed to be l~C-depleted relative to methane produced from the decay of C4 vegetation (Chanton and Smith, 1993). Across what kind of spatial and temporal gradients can one expect to find transitions in the methane production mechanism? Methane is produced primarily in flooded basins and sedimentary environments; in these environments, the production of acetate and its subsequent utiliza- tion are generally greater in the upper portion of the peat or sediment column than in deeper layers (Whiticar, 1999 and references therein).
Details
-
File Typepdf
-
Upload Time-
-
Content LanguagesEnglish
-
Upload UserAnonymous/Not logged-in
-
File Pages21 Page
-
File Size-