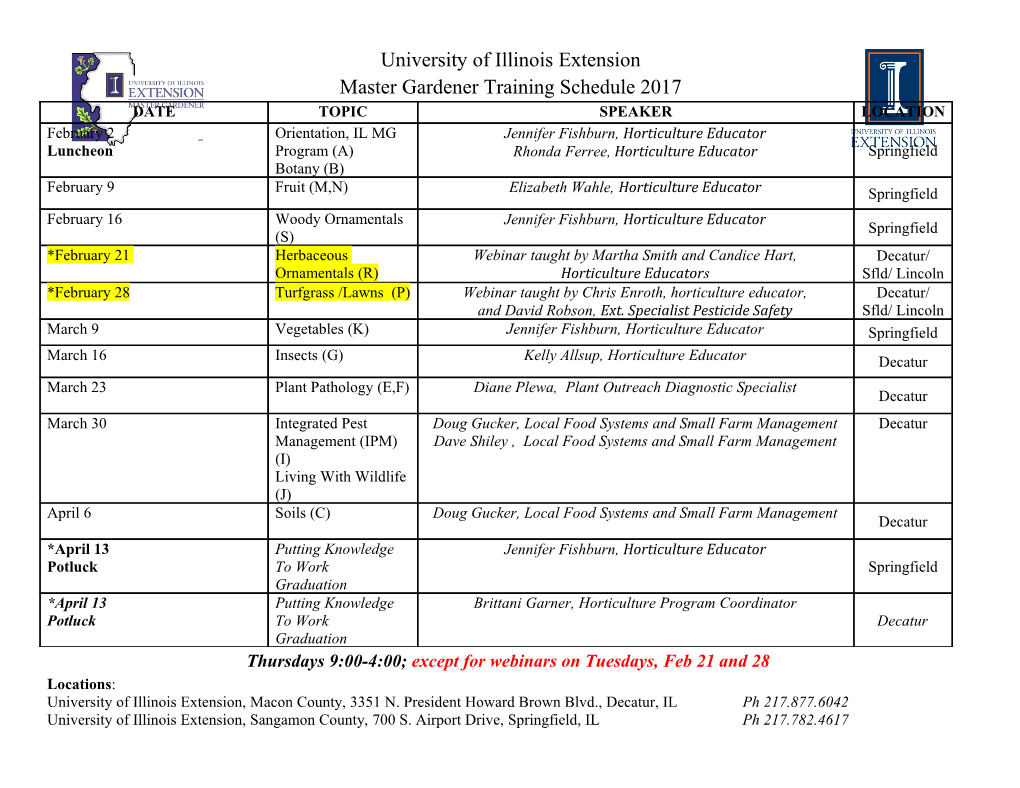
applied sciences Article Experimental Investigations on Laser Ablation of Aluminum in Sub-Picosecond Regimes Katarzyna Garasz * and Marek Kocik Centre for Plasma and Laser Engineering, Institute of Fluid Flow Machinery, Polish Academy of Sciences, Fiszera 14, 80-231 Gda´nsk,Poland; [email protected] * Correspondence: [email protected]; Tel.: +48-5225117 Received: 15 October 2020; Accepted: 10 December 2020; Published: 12 December 2020 Abstract: Due to high power and ultrashort pulses, femtosecond lasers excel at (but are not limited to) processing materials whose thicknesses are less than 500 microns. Numerous experiments and theoretical analyses testify to the fact that there are solid grounds for the applications of ultrafast laser micromachining. However, with high costs and complexity of these devices, a sub-picosecond laser that might be an alternative when it comes to various micromachining applications, such as patterns and masks in thin metal foils, micro-nozzles, thermo-detectors, MEMS (micro electro-mechanical systems), sensors, etc. Furthermore, the investigation of sub-picosecond laser interactions with matter could provide more knowledge on the ablation mechanisms and experimental verification of existing models for ultrashort pulse regimes. In this article, we present the research on sub-picosecond laser interactions with thin aluminum foil under various laser pulse parameters. Research was conducted with two types of ultrafast lasers: a prototype sub-picosecond Yb:KYW laser (650 fs) and a commercially available femtosecond Ti:S laser (35 fs). The results show how the variables such as pulse width, energy, frequency, wavelength and irradiation time affect the micromachining process. Keywords: sub-picosecond regime; femtosecond pulses; precise micromachining; laser ablation; ablation threshold; micromachining of aluminum 1. Introduction A scientific goal of this study is a better understanding of the physical phenomena and identification of ablation mechanisms in sub-picosecond regimes. A practical goal is to explore and enable sub-picosecond (i.e., in the range of several hundred of femtoseconds) lasers as an alternative to both femtosecond and picosecond systems in high precision micromachining processes. Even though there are already femtosecond industrial lasers able to work for production environments, given the full advantages of material micromachining without the necessity of post-processing tools, they are not considered the first choice for many manufacturers. Still, the complex and unstable nature of femtosecond systems undermines their reliability and efficiency in commercial use, in contrast to the picosecond laser systems [1]. An effective micromachining process depends on the laser beam parameters, such as pulse duration, wavelength, energy, frequency or irradiation time, as well as target material properties [2]. These parameters affect the course of laser ablation, which engages different mechanisms than longer, i.e., picosecond and nanosecond, pulses [3,4]. We found this region between picosecond and femtosecond laser pulses interesting for further investigation, since both short and long pulse interactions with matter may appear. Most of commercial femtosecond lasers offer the pulse length of tens of femtoseconds. In our research, we used a popular 35 fs Ti:S laser and a 650 fs prototype Yb:KYW laser. Both offered adjustable wavelength (UV/VIS/IR) and frequency. The pulse energy ranges were picked in the manner to make Appl. Sci. 2020, 10, 8883; doi:10.3390/app10248883 www.mdpi.com/journal/applsci Appl. Sci. 2020, 10, 8883 2 of 8 the results maximally comparable for both lasers. This approach resulted in a complex parametric study, whereas previous research mainly focused on one or two selected parameters [2–5]. 2. Theoretical Background The discovery of the chirped pulse amplification (CPA) method [6] and, in consequence, ultrafast lasers, opened a new field for both fundamental and applied research. Ablation that occurs during the athermal state of the sample in the dynamics of the pulse absorption, commonly known as cold ablation, became one of the most promising applications of ultrafast lasers. The process occurs within a time range of tens of nanoseconds and differs significantly for long and ultrashort laser pulses. Most of the experiments are carried out with Gaussian beams and just above the ablation threshold. The ablation process involves stages such as: energy absorption, energy transfer to the lattice and material removal. After the pulse energy is deposed into the material, the excitation of electrons from the valance to the conduction band occurs, as well as the free carrier absorption. With significant beam intensity, either multiphoton or avalanche ionization appears. Nonlinear absorption is therefore a dominant mechanism in ultrashort pulse regimes [7]. The occurrence of the complex processes during laser interactions with matter is almost simultaneous, which makes it difficult to determine the contribution of each one. Depending on the material, on a timescale from hundreds of fs to a few ps, the energy transfer from electrons to the lattice takes place. Hence, the non-equilibrium state of the electrons and the lattice are best portrayed by a two-temperature model [8]. The transfer of energy results in an accelerated thermal or non-thermal melting [9]. Since the mass transport happens in much longer timescales than the non-thermal or thermal melting, the melted parts of material are left at a hot, near solid state. Subsequently, the hydrodynamic expansion of the ablated material begins, on a timescale of a few hundred ps after the initial excitation [10]. Despite the numerous investigations on the fundamentals of laser material removal, those in the femtosecond regime are still argued. To calculate the estimated ablation threshold in this study, several theoretical models were considered [11–13]. We found the following solution proposed by Gamaly et al. to be the most adequate: 3 ληa F = (" + "esc) (1) th 8 b 2π According to Equation (1), in case of metals, the ablation threshold’s Fth definition is based on the condition that the electron energy must reach the value equal to the sum of the atomic binding energy "b and the work function "esc; ηa is electron density [11]. By this definition, the threshold values for the aluminum samples used in our research have been calculated for each wavelength λ. The results are presented in Table1. Table 1. Calculated values of the aluminum ablation threshold, for different wavelengths. Wavelength (nm) 1030 800 532 345 266 Ablation threshold (J/cm2) 1.36 1.06 0.70 0.46 0.35 3. Experimental Setup The micromachining of aluminum samples was conducted with two different lasers, a sub-picosecond Yb:KYW (Lab-designed at the Institute of Physical Chemistry PAS, Warsaw, Poland, 2010) and a femtosecond Ti:S laser (Spectra-Physics, Santa Clara, CA, USA, 2012). The parameters are presented in Table2. Appl. Sci. 2020, 10, 8883 3 of 8 Table 2. The specifications of the two ultrafast lasers used in the experimental setup. Parameter Sub-Picosecond Femtosecond Appl. Sci. 2020, 10, x FOR PEER REVIEW 3 of 9 Laser type Yb:KYW Ti:S LaserPulse type duration (fs) 650Yb:KYW 35 Ti:S PulseAvailable duration wavelength (fs) (nm) 345–1030650 240–2600 35 Available wavelengthFrequency (kHz) (nm) 100–400345–1030 1–10 240–2600 FrequencyPulse (kHz) energy (µJ) 140100–400 6000 1–10 Pulse energy (µJ) 140 6000 For the first laser, 1030 nm and 345 nm wavelengths were made available for the micromachining For the first laser, 1030 nm and 345 nm wavelengths were made available for the processes. For the second one, three different wavelengths from the UV, VIS and IR range were picked, micromachining processes. For the second one, three different wavelengths from the UV, VIS and IR as well as being used for the calculations in Table1. range were picked, as well as being used for the calculations in Table 1. Figure1 shows that, as the laser beam passes through the optical collimator and focusing system, Figure 1. shows that, as the laser beam passes through the optical collimator and focusing consisting of mirrors and lenses adequate for the applied laser wavelength, it is then transferred to the system, consisting of mirrors and lenses adequate for the applied laser wavelength, it is then high-precision positioning system, based on XY linear motors. [14] transferred to the high-precision positioning system, based on XY linear motors. [14] Figure 1. The experimental setup for ultrafast laser micromachining. 4. Results and Discussion Figure 1. The experimental setup for ultrafast laser micromachining. As it was previously stated, ablation mechanisms strongly depend on the laser beam parameters [2]. The4. Results methodology and Discussion of the conducted experiments is presented in Table3. In the materials undergoing laser micromachining, there are various markers that can be connected As it was previously stated, ablation mechanisms strongly depend on the laser beam parameters to the dynamical mechanisms and the ablation threshold. Among them, there are crater depth and [2]. The methodology of the conducted experiments is presented in Table 3. width, local changes in material structure, cracks, surface debris, and colour change caused by melting, to mention a few. To analyse someTable of those 3. The features, summary we of usedthe experiment a Nikon Eclipse cases. metallographic microscope (E100, Nikon Corporation, Tokyo, Japan, 2006) and a 3D Hirox KH8700 microscope (Hirox Co. Ltd., Tokyo,
Details
-
File Typepdf
-
Upload Time-
-
Content LanguagesEnglish
-
Upload UserAnonymous/Not logged-in
-
File Pages8 Page
-
File Size-