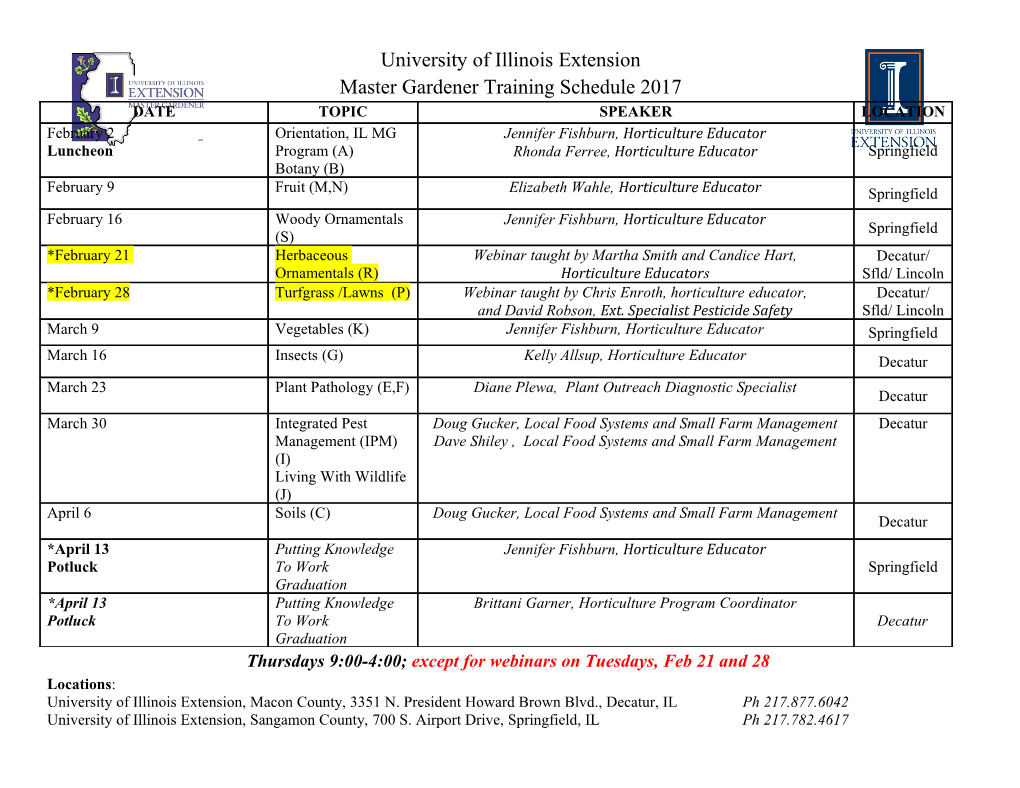
UNIVERSITÀ DEGLI STUDI DI NAPOLI FEDERICO II Scuola Politecnica e delle Scienze di Base Corso di Dottorato in Ingegneria Industriale (XXIX Ciclo) Dipartimento di Ingegneria Industriale Tesi di Dottorato Modelling and Acoustic Optimization of an Intake System for an Internal Combustion Engine Danilo D’Agostino COORDINATORE Ch.mo Prof. Ing. Michele Grassi TUTORI Ch.mo Prof. Ing. Fabio Bozza Ing. Daniela Siano Anno 2017 To my parents… I Acknowledgements I am very grateful to my friends and colleagues Roberto Aiello and Maria Antonietta Panza for the very interesting discussions on sociological and scientific topics and with whom I have shared three incredible, unbelievable years. Their presence has been very important in this path of both personal and professional growth. My sincere thanks go to PhD Luigi Teodosio for his friendship and for the very valuable time we spent together talking about various matters. Thanks to my supervisors, Daniela Siano, who gave me the opportunity to start such journey in the research environment, and prof. Fabio Bozza for his technical teachings and his support related to the performed activities. Thanks to all the people I have met during the PhD, for their support, encouragement and suggestions. Last but not the least, a special thank goes to my family and friends, for their unconditioned, priceless love and presence. Thanks to Claudia, for being as she is and for her support and understanding during all these years. II Abbreviations CAE Computer Aided Design 1D One-Dimensional 3D Three-Dimensional TL Transmission Loss SI engine Spark Ignition engine GA Genetic Algorithm IPFM Interior Penalty Function Method EPFM Exterior Penalty Function Method FDM Feasible Direction Method FE Finite Element OSPL Overall Sound Pressure Level IL Infinite Element NR Noise Reduction PDE Partial Differential Equation CAD Computer Aided Design IE Infinite Element TM Transfer Matrix FSI Fluid-Structure Interaction CFD Computational Fluid Dynamic IVO Inlet Valve Opening VVA Variable Valve Actuator WOT Wide Open Throttle RANS Reynolds Average Navier-Stokes OASPL Overall A-weighted Sound Pressure Level III SPL Sound Pressure Level IV Contents Introduction………………………………………………………………………………………... 1 References……………………………………………………………………………..……….........8 Chapter 1: Basics of Fluid Flow…………………………………………………………………... 9 1.1 The divergence theorem……………………………………………………………………...9 1.2 Conservation equations…………………………………………………………………… 11 1.2.1 Reynolds Transport theorem…………………………………………………………17 1.2.2 Conservation of mass………………………………………………………………… 19 1.2.3 Conservation of momentum………………………………………………………….. 20 1.2.3.1 Stokes’ law….............................................................................................................23 References…………………………………………………………………………………….........26 Chapter 2: The Concept of Sound and its Propagation………………………………………... 27 2.2 The Sound……………………………………………………………………………………27 2.1.1 Frequency domain study of sound…………………………………………………….29 2.2 Sound propagation…………………………………………………………………………..33 2.2.1 1-D homogeneous classical wave equation……………………………………………34 2.2.1.1 Solution of the 1-D homogeneous classical wave equation……………………... 36 2.2.2 Homogeneous classical wave equation and solution……………………………….. 40 2.2.3 3-D propagation in tubes…………………………………………………………….. 43 2.3 Sound propagation in moving media……………………………………………………... 46 2.3.1 The convective wave equation and solution………………………………………….46 References…………………………………………………………………………………….........50 Chapter 3: Basics of Sound Propagation in Duct………………………………………………..51 3.1 Basic theory of acoustic filters……………………………………………………………...52 3.1.1 Specific acoustic impedance of an uniform tube…………………………………….53 3.1.2 Radiation impedance………………………………………………………………….55 3.1.3 A lumped inertance……………………………………………………………………60 3.1.4 End correction………………………………………………………………………... 61 3.1.5 A lumped compliance………………………………………………………………….62 3.1.6 Acoustic resonators…………………………………………………………………….63 3.1.6.1 Extended-tube (quarter-wave) resonator………………………………………...64 3.1.6.2 Helmholtz resonator……………………………………………………………….67 3.1.6.3 Concentric hole-cavity resonator…………………………………………………68 3.2 Acoustic filter performance parameters…………………………………………………...69 3.2.1 Insertion Loss………………………………………………………………………….70 3.2.2 Transmission Loss……………………………………………………………………. 72 3.2.3 Noise Reduction………………………………………………………………………..73 3.2.4 Simple area discontinuities…………………………………………………………... 76 V 3.3 Introduction to the study of porous materials…………………………………………… 78 3.3.1 Equivalent fluid models……………………………………………………………….81 References…………………………………………………………………………………….........83 Chapter 4: Finite Element Method in Acoustics…………………………………………………84 4.1 Problem statement…………………………………………………………………………..85 4.2 Galerkin procedure…………………………………………………………………………87 4.3 Convergence of the model…………………………………………………………………. 93 4.4 Modelling exterior acoustic problems…………………………………………………….96 References…………………………………………………………………………………….........99 Chapter 5: Acoustic Performance Analysis……………………………………………………..100 5.1 Transfer Matrix Method…………………………………………………………………. 101 5.1.1 Experimental evaluation of the TL: the impedance tube technology……………..105 5.1.1.1 Transfer Matrix formulation: the two-load method…………………………...107 5.2 Experimental procedure…………………………………………………………………. 108 5.3 Numerical procedure…………………………………………………………………….. 113 5.3.1 CAD preparation and FE models creation………………………………………... 113 5.3.1.1 Numeric purely acoustic models……………………………………………….. 116 5.3.1.2 Numeric structural-acoustic model……………………………………………..121 5.4 Experimental vs Numerical comparison: model validation…………………………… 126 References…………………………………………………………………………………….......132 Chapter 6: Gas-dynamic Noise Analysis………………………………………………………...133 6.1 Experimental analysis……………………………………………………………………. 135 6.2 Numerical analysis………………………………………………………………………...137 6.2.1 One-dimensional analysis…………………………………………………………....137 6.2.2 Three-dimensional acoustic analysis………………………………………………..144 References…………………………………………………………………………………….......154 Chapter 7: Aerodynamic Generation of Sound………………………………………………...155 7.1 The inhomogeneous wave equation and the Green’s function…………………………..157 7.2 Multipole expansion of sound fields……………………………………………………....161 7.2.1 Monopole source…………………………………………………………………….. 165 7.2.2 Dipole source………………………………………………………………………… 168 7.2.3 Quadrupole source………………………………………………………………….. 175 7.3 Lighthill’s Aeroacoustic Analogy………………………………………………………... 178 7.3.1 Modelling approach………………………………………………………………… 180 7.3.1.1 Monopole source term…………………………………………………………...183 7.3.1.2 Dipole source term……………………………………………………………….185 7.3.1.3 Quadrupole source term……………………………………………………….. 187 7.4 Introduction to turbulence modelling…………………………………………………….190 7.4.1 Reynolds Stress Equation…………………………………………………………….194 7.4.2 풌 − 휺 turbulence model……………………………………………………………...196 VI References…………………………………………………………………………………….......200 Chapter 8: Geometric Optimization…………………………………………………………….201 8.1 Transmission Loss enhancement…………………………………………………………205 8.2 Gas-dynamic noise Analysis………………………………………………………………220 8.2.1 Problem statement…………………………………………………………………... 220 8.2.2 Analysis process……………………………………………………………………... 224 8.2.2.1 One-dimensional CFD analysis………………………………………………… 224 8.2.2.2 Three-dimensional CFD analysis………………………………………………..228 8.2.2.3 Three-dimensional acoustic analysis…………………………………………... 232 References…………………………………………………………………………………….......247 Chapter 9: Conclusions and Future Developments…………………………………………….248 VII Introduction Introduction Noise is recognized as having a significantly adverse effects on our everyday lives, including interference with communication, sleep disturbance, annoyance responses, performance effects as well as health through psycho-physiological effects. As an everyday example, noise induced annoyance is a very important problem occurring when people are constantly exposed to a noise source, regardless of its intensity. Such evidence has been recognized by product designers engineers within the world’s most innovative and successful companies, which try to incorporate effective noise mitigation measures into their product design process. Such new project aspect has become extremely important in the automotive industry too and it is possible to state that there is a strong competition among automotive manufacturers to generally reduce the radiated noise levels. In fact, nowadays, the concept of client perception quality of a vehicle, which represents the prior target behind the production strategies of cars manufacturers, is quite changed with respect to a dozen of years ago. In particular, among the factors identifying the quality of a vehicle, the concept of delivered sound represents a critical aspect that manufacturers are focusing on, in order to be more competitive on the global market. As matter of fact, poor sound quality can give the impression of poor vehicle build quality or, for a customer point of view, can give the idea that there is something wrong with the operations of the vehicle, as if something is not working properly and is going to break. More precisely, the term sound quality refers to whether sounds are pleasant or annoying. For example, if a properly tuned engine noise is something that may be appreciated by customers, on the other hand it may strongly reduce the global comfort. Consequently, every vehicle component is not only designed in order to ensure the achievements of its specific goal but also considering the impact on the vehicle’s overall sound quality.
Details
-
File Typepdf
-
Upload Time-
-
Content LanguagesEnglish
-
Upload UserAnonymous/Not logged-in
-
File Pages259 Page
-
File Size-