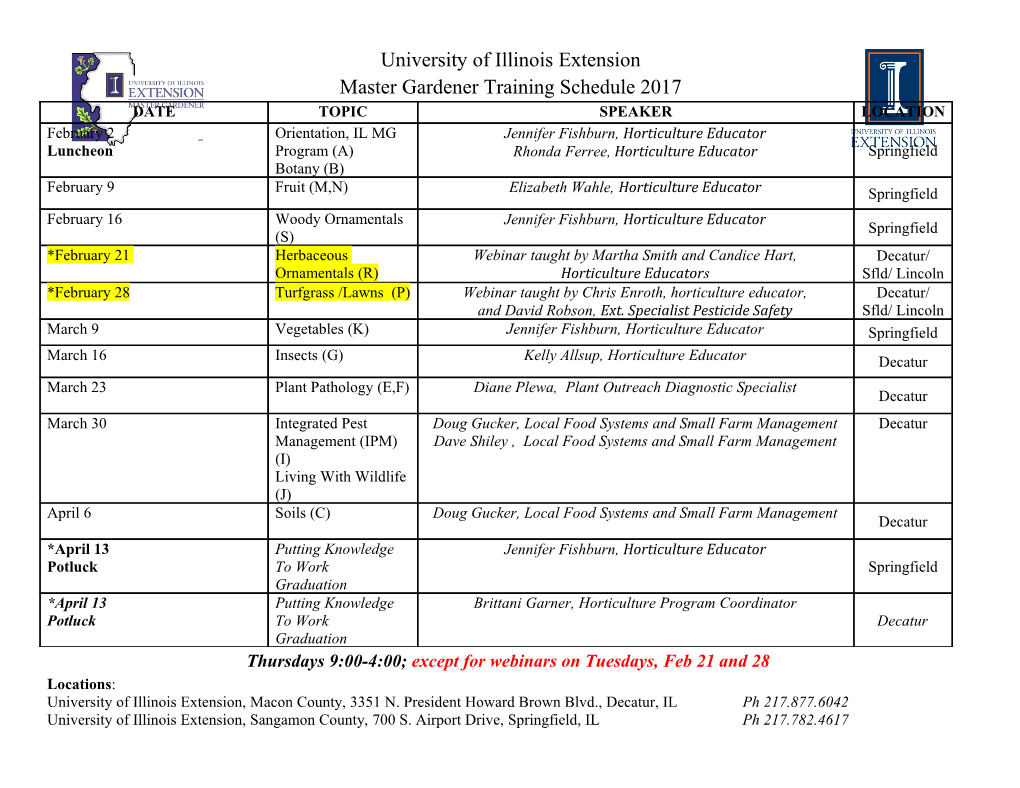
276 C. LULEY-GOEDL et al.: Biocatalytic Production of a-Glucosylglycerol, Food Technol. Biotechnol. 48 (3) 276–283 (2010) ISSN 1330-9862 review (FTB-2486) Biocatalytic Process for Production of a-Glucosylglycerol Using Sucrose Phosphorylase Christiane Luley-Goedl, Thornthan Sawangwan, Mario Mueller, Alexandra Schwarz and Bernd Nidetzky* Institute of Biotechnology and Biochemical Engineering, Graz University of Technology, Petersgasse 12, A-8010 Graz, Austria Received: March 25, 2010 Accepted: April 28, 2010 Summary Glycosylglycerols are powerful osmolytes, produced by various plants, algae and bac- teria in adaptation to salt stress and drought. Among them, glucosylglycerol (2-O-a-D-glu- copyranosyl-sn-glycerol; GG) has attracted special attention for its promising application as a moisturizing agent in cosmetics. A biocatalytic process for the synthesis of GG as in- dustrial fine chemical is described in which sucrose phosphorylase (from Leuconostoc me- senteroides) catalyzes regioselective glucosylation of glycerol using sucrose as the donor substrate. The overall enzymatic conversion, therefore, is sucrose+glycerol®GG+D-fructose. Using a twofold molar excess of glycerol acceptor in highly concentrated substrate solu- tion, GG yield was 90 % based on ³250 g/L of converted sucrose. Enzymatic GG produc- tion was implemented on a multihundred kg-per-year manufacturing scale, and a com- mercial product for cosmetic applications is distributed on the market under the name Glycoin®. Technical features of the biotransformation that were decisive for a successful process development are elaborated. Stabilization of proteins is another interesting field of application for GG. Key words: glucosylglycerol, sucrose phosphorylase, transglucosylation, glycerol, Glycoin®, protein stabilization, osmolytes tion of proteins and the stabilization of supramolecular Introduction biological structures like those of lipid membranes. Microbial life under extremes of temperature, osmo- Glycosylglycerols are powerful osmolytes that are tic pressure and pH is facilitated by the intracellular ac- produced by various plants, algae, and bacteria in adap- cumulation of a diverse class of small molecules that are tation to salt stress and drought (9). Among them, 2-O- often referred to as compatible solutes (1–4). A conserved -a-D-glucopyranosyl-sn-glycerol (GG; Scheme 1), which characteristic for many of these compounds is a glyco- is the main compatible solute in photosynthetic bacteria sidic chemical structure (Scheme 1). Compatible solutes (10), has attracted special attention for its promising (also termed osmolytes) are found ubiquitously in na- application as a moisturizing agent in cosmetics (11–13), ture where they serve a conserved function in protecting but also for use as low-calorie sweetener that does not cells against high salt concentration, extremes of tempe- cause tooth decay (14). Possible therapeutic applications rature and other forms of external stress. Their physio- of GG that are inferred from its ability to stabilize pro- logical effectiveness (5–7) and technological performance teins and cells are currently being evaluated (15,16). (3,8) can be traced back to the ability to regulate the cel- However, the development of industrial applications for lular water balance and to the prevention of denatura- GG (and related carbohydrate-based compatible solutes) *Corresponding author; Phone: ++43 316 873 8400; Fax: ++43 316 873 8434; E-mail: [email protected] C. LULEY-GOEDL et al.: Biocatalytic Production of a-Glucosylglycerol, Food Technol. Biotechnol. 48 (3) 276–283 (2010) 277 OH 44 (20). The enzyme has been characterized from a re- HO OH HO HO latively small number of bacterial species, namely Bifido- OH bacterium adolescentis (21), Leuconostoc mesenteroides (20,22– HO HO O O O HO 24), Pseudomonas saccharophila (25–27) and Streptococcus mu- O O HO tans (28–30). However, genes encoding putative sucrose OH HO O HO OH O O phosphorylases have been detected in many other spe- HO HO OH O cies, mainly in members of the bacterial class of Bacillus. HO MG HO MGA trehalose The enzyme is about 500 amino acids long and was found OH HO HO HO to occur as a functional monomer or dimer. Its activity is OH not dependent on cofactors or cosubstrates. A crystal HO HO O O O structure has been determined for the homodimeric su- O O HO OH HO HO crose phosphorylase from B. adolescentis (31). The enzyme OH O HO OH O O adopts the canonical (b/a) -barrel fold (Fig. 1a) of gly- HO HO OH 8 O coside hydrolases and transglycosidases of family GH13 GG GGA sucrose HO HO (the 'a-amylase family') of the glycoside hydrolase fami- Scheme 1. Chemical structures of mannosylglycerol (MG), lies. Therefore, in spite of its EC categorization as a gly- mannosylglycerate (MGA), glucosylglycerol (GG), glucosylgly- cosyltransferase, sucrose phosphorylase is structurally cerate (GGA), a,a-trehalose and sucrose related to glycoside hydrolases. The active site residues, a triad of two aspartic acids and one glutamic acid, are highly conserved in both sequence and structure (Fig. was severely restricted by compound availability. Previ- 1b). ously reported synthetic procedures were not technolo- Biochemical studies of sucrose phosphorylase go back gically mature due to difficult problems of insufficient in time to the 1940s. The enzyme was first discovered in yield, selectivity, productivity, or a combination of these L. mesenteroides (32) and P. saccharophila (33). Mieyal and 14,17–19 major limitations ( ). We have described a new Abeles (34) summarized evidence from early mechani- biocatalytic process which overcomes the chemical and stic work on sucrose phosphorylase that led to the per- technological challenges of the production of stereoche- ceptive proposal of a double displacement-like reaction mically pure GG as an industrial chemical. mechanism involving a catalytically competent covalent enzyme intermediate. A b-configured O-glycosidic link- Sucrose Phosphorylase age between the glucosyl moiety and a carboxylate side chain from Asp/Glu was demonstrated (35). The pro- Sucrose phosphorylase (sucrose:phosphate a-D-gluco- posed covalent intermediate has now also been seen cry- syltransferase; EC 2.4.1.7) catalyzes the reversible conver- stallographically (36). Residues involved in catalysis were sion of sucrose (a-D-glucopyranosyl-1,2-b-D-fructofurano- probed and their proposed function confirmed by kine- side) and phosphate into a-D-glucopyranosyl phosphate tic characterization of relevant site-directed mutants (37– (G1P) and D-fructose. The equilibrium constant for the 39). The catalytic mechanism of sucrose phosphorylase phosphorylase reaction at pH=7.0 and 30 °C is approx. is summarized in Scheme 2. Fig. 1. Sucrose phosphorylase structure: a) three-dimensional fold of the subunit of sucrose phosphorylase from B. adolescentis deri- ved from the crystal structure of the dimeric enzyme (PDB entry 1r7a), b) close-up structure of the active site in the b-glucosyl enzyme intermediate of the phosphorylase (PDB entry 2gdv, molecule A). Residues of the catalytic triad, Asp192 (nucleophile; glu- cosylated in the structure), Glu232 (acid–base), and Asp290 (transition state stabilizer) are indicated. Partial multiple sequence align- ment of primary structures of sucrose phosphorylase enzymes from B. adolescentis (BaSPase, GenBank AAO33821), L. mesenteroides (LmSPase, GenBank BAA14344), P. saccharophila (PsSPase, GenBank AAD40317), and S. mutans (SmSPase, GenBank AAA26937). The alignment was performed with the Vector NTI program using the AlignX-modul with the blosum62mt2 scoring matrix 278 C. LULEY-GOEDL et al.: Biocatalytic Production of a-Glucosylglycerol, Food Technol. Biotechnol. 48 (3) 276–283 (2010) HO O HO HO O 2- D-fructose O HPO4 HO O HO HO O O O O O OH O HO O HO HO O OH P HO HO HO _ O O H O H OH O OH O O O O O Scheme 2. Proposed catalytic mechanism for sucrose phosphorylase. The enzymatic reaction proceeds in two catalytic steps via aco- valent enzyme intermediate. For reasons of clarity, only the catalytic nucleophile (top) and the general acid–base (bottom) are shown Sucrose phosphorylase is known to catalyze three Biocatalytic Synthesis of GG types of overall reaction, as shown in Scheme 3: gluco- syl transfer to and from phosphate, often termed phos- The enzymatic reaction used for the synthesis of GG phorolysis and synthesis; hydrolysis; and transglucosyl- is shown in Scheme 4. Sucrose phosphorylase promotes ation. Hydrolysis and transglucosylation are reactions of transglucosylation in two steps where the b-glucosyl-en- the glucosylated phosphorylase when phosphate is lack- zyme intermediate formed in the first half-reaction (en- ing. Hydrolysis occurs at a rate of ³50 times more slowly zyme glucosylation) is intercepted by the 2-OH of glyce- than glucosyl transfer to phosphate. External acceptors rol in the second half-reaction to yield the a-configured differ in reactivity towards glucosylation by the phos- glucosidic product (enzyme deglucosylation). Hydro- phorylase (40). lysis of the intermediate potentially competes with group transfer to the acceptor substrate. Furthermore, hydro- a Sucrose, G1P and -D-glucopyranosyl fluoride (G1F) lysis of a product would constitute an undesired second- are highly reactive donor substrates for the enzyme. The ary reaction catalyzed by the enzyme (see later). range of acceptor substrates is broad (34,41), defining Glucosyl transfer from sucrose to glycerol was char- the scope for sucrose phosphorylase as a useful trans-
Details
-
File Typepdf
-
Upload Time-
-
Content LanguagesEnglish
-
Upload UserAnonymous/Not logged-in
-
File Pages8 Page
-
File Size-