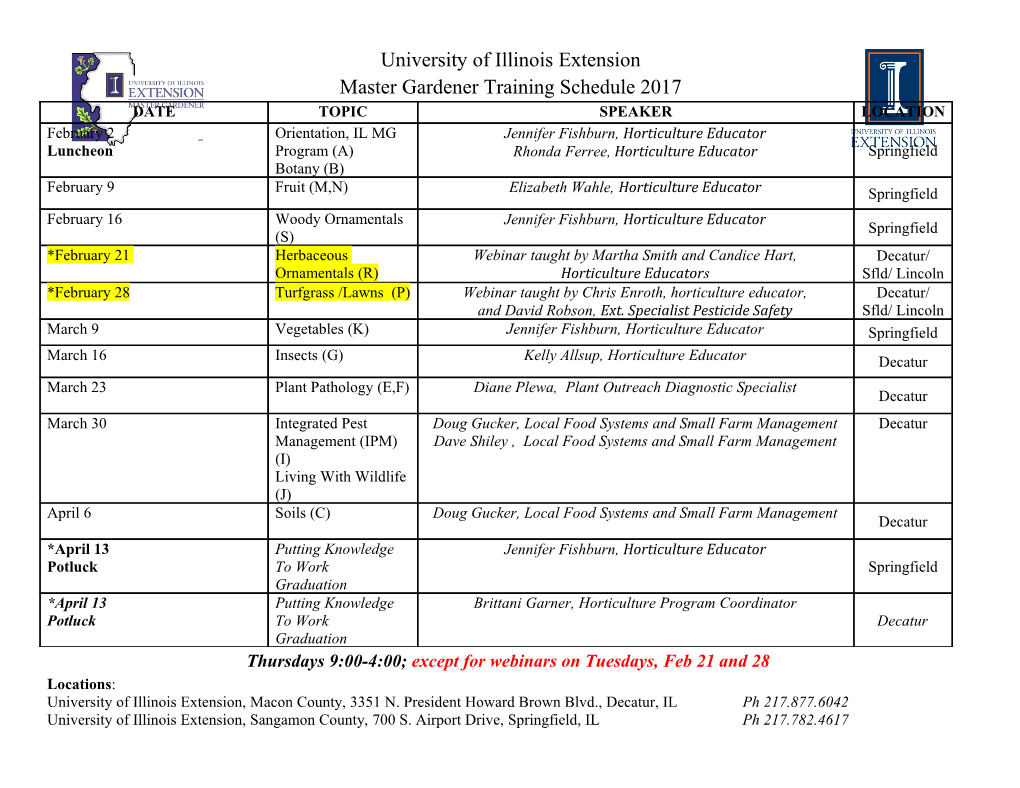
The Global Phosphorus Cycle Continental Leached / Eroded Terrestrial Rivers / Wind crust biosphere Ocean Subducted / Cycled by Uplifted marine biota & Marine buried sediments • P biogeochemical cycling in the different reservoirs • Inventories & interactions with other biogeochemical cycles Why phosphate? World Ocean Atlas, 2001 • Limiting nutrient on geologic timescales (Tyrell, 1999) • Need to constrain P budget • Understand the factors that influence its oceanic inventory Why phosphate? Are oceanic phytoplankton P or N limited? ‘Geochemists' viewpoint’ : nitrogen can be "topped up" from the atmosphere by N2 fixation; phosphorus has no comparable sources or biological pathways ‘Biologists' viewpoint’ : observational and experimental work finds natural assemblages of phytoplankton are more N- stressed than P-stressed Levitus Climatology P reservoirs Atmosphere 0.9 10-3 0.03 0.15 < 0.01 Land Biota: 102 Surf. Ocean 33 Ocean Biota: 0.9 102 32.7 4 • Atmosphere not a 2 2 Land: 6.5 103 0.2 0.3 significant reservoir Deep Ocean 0.4 0.7 3 • P only occurs in 1 Minable P 2.8 10 3 102 oxidation state (+5), 0.6 0.1 moles/y orthophosphate 8 1.3 10 Crust + Sediment 3- PO4 Inventories = 1012 moles (bold) Fluxes = 1012 moles / year Figure by MIT OCW. P in the lithosphere Atmosphere 0.9 10-3 • Largest reservoir 0.03 0.15 < 0.01 2 Land Biota: 10 Surf. Ocean 33 Ocean Biota: 0.9 102 32.7 4 2 2 • >95% crustal P = Apatites Land: 6.5 103 0.2 0.3 Deep Ocean Ca (PO ) (F,OH,Cl) 0.4 0.7 10 4 6 2 3 Minable P 2.8 10 3 102 F = fluorapatite 0.6 0.1 moles/y 1.3 108 Crust + Sediment OH = hydroxyapatite Inventories = 1012 moles (bold) Fluxes = 1012 moles / year Cl = chlorapatite Figure by MIT OCW. • Most common apatite mineral = carbonate fluorapatite Ca10(PO4)6-x(CO3)x(F)2+x Carbonate partially substitutes for 3- 2- phosphate (PO4 vs. CO3 ) • Not only in igneous rocks… Apatite minerals • Hydroxyapatite ⇒ produced by biota • Sedimentary deposits ⇒ phosphorite (18-40% P be weight) = fertilizer • Fluorapatite used in fluoridated water and toothpastes Anthropogenic processes may change the distribution of the P inventories P on land & in the biota • Weathering and erosion of uplifted rocks produce dissolved (reactive) and particulate (inert) forms of phosphate • Dissolved P entirely tetrahedral phosphate ions − + 2− + 3− + H PO3 H 4↔ 2 PO+ 4 H ↔ +4 H2 HPO ↔ PO4 3 + H DISTILLED WATER 100 H PO - 2- 2 4 HPO4 50 H3PO4 0 Acid / base equilibria 2 3 4 5 6 7 8 9 10 - 34.8 % SEAWATER Freshwater pH ~6 – 7 ⇒ H2PO4 100 H PO- 2- 3- 2 4 HPO4 PO4 2- 50 Ocean pH ~ 8.2 ⇒ HPO4 H3PO4 % OF TOTAL INORGANIC PHOSPHATE TOTAL % OF 0 2 3 4 5 6 7 8 9 10 pH Distribution of phosphoric acid species as a function of pH in distilled water and seawater. (Atlas, 1975). Figure by MIT OCW. P on land & in the biota • Dissolved (reactive) is taken up & cycled by terrestrial biota • Produces organophosphates (Porg), phosphate esters Most important P-containing organic molecules are DNA, RNA, ATP, & phospholipids Chemical Structure of RNA, DNA, and ATP. Phospholipids Image removed due to copyright restrictions. ⇒ phosphate esters produced with fatty acids containing hydrophobic C chains (important constituent of cell membranes Phosphate CH3 O + H3CN CH2 CH2 OP O CH2 CH3 O O H H H H H H H H H H H H H H H H H Choline HC O O C C C C C C C C C C C C C C C C C H H H H H H H H H H H H H H H H H H O H H H H H H H H H H H H H H H H H H2COO C C C C C C C C C C C C C C C C C H H H H H H H H H H H H H H H H H H Polar Glycerol Fatty head group Figure by MIT OCW. backbone acid chains P on land & in the biota • Dissolved phosphate concentrations are low in soil: Adsorbed onto clays, Fe and Al oxides • Terrestrial systems are often P limited Plants ⇑ P availability via chelating compounds • C / P (moles / moles) marine organisms ~ 106 (Redfield) • C / P terrestrial plants >> 106 • Terrestrial plants contains less P per mole C due to: Higher proportion of structural C-rich and P-poor molecules, carbohydrates and ligins P on land & in the biota Turnover time of land biota: Atmosphere 0.9 10-3 0.03 0.15 < 0.01 1014 moles / 2 x 1012 2 Land Biota: 10 Surf. Ocean 33 Ocean Biota: 0.9 102 32.7 4 -1 2 2 moles yr Land: 6.5 103 0.2 0.3 Deep Ocean ~ 50 years 0.4 0.7 3 Minable P 2.8 10 3 102 0.6 0.1 moles/y Retention time of 1.3 108 Crust + Sediment reactive & eroded P Inventories = 1012 moles (bold) on land: Fluxes = 1012 moles / year 6.5 x 1015 moles / 0.7 Figure by MIT OCW. x 1012 moles yr-1 ~ 9000 years P transport to the ocean - Aeolian • Small source due to Aeolian transport of dust (no gaseous forms of P) Total dust flux x Mean [P] in aerosols x solubility Mahowald et al., 2005a = Total Aeolian P flux Dust input to the ocean is highly variable: spatially, seasonally (rainfall & transport patterns), glacial-interglacial, episodic (wind speed) P transport to the ocean - Aeolian Total dust flux x Mean [P] in aerosols x solubility = Total Aeolian P flux Solubility of dust P ⇒ 21 – 51% (Graham and Duce, 1982) Aeolian input of reactive P ⇒ 0.01 – 0.02 x 1012 moles yr-1 Aeolian input <<< fluvial input Aeolian input of P (109 g/year) Even in oligotrophic gyres: North Pacific 500 South Pacific 41 estimates of Aeolian input account North Atlantic 230 for less then 1% of new production South Atlantic 25 North Indian 110 South Indian 46 Global Total 950 Factor of 2-3 uncertainty P transport to the ocean - Fluvial • Rivers are the primary source of P for the oceans • 90-95% of P in river is in particles: Inert minerals directly eroded from continental crust Fe and Al oxides (P adsorbed as oxides & clay) • In estuaries reactive P can be: Desorbed from Fe oxides and clays Removed into estuarine sediments as Porg after biological uptake, by salinity induced flocculation of Fe- humic river colloids • Small amounts of P desorption can greatly ⇑ our estimates of the fluvial input of bioavailable phosphate • Current estimates 0.03 – 0.15 x 1012 moles yr-1 P cycling in seawater DISTILLED WATER 100 H PO - 2- 2 4 HPO4 In seawater dissolved 50 H3PO4 inorganic phosphorus 0 2 3 4 5 6 7 8 9 10 primarily HPO 2- 34.8 % SEAWATER 4 100 H PO- 2- 3- 2 4 HPO4 PO4 50 Ion pairs are formed with H3PO4 % OF TOTAL INORGANIC PHOSPHATE TOTAL % OF 0 2 3 4 5 6 7 8 9 10 the major cations in pH Distribution of phosphoric acid species as a function of pH in distilled water and seawater. seawater (Atlas, 1975). Figure by MIT OCW. + 2- MgH2PO4 - 0.1% HPO4 (free) + NaHPO - CaH2PO4 - 0.01% 4 28.7 % 15 % + NaH2PO4 - 0.1% H PO- (free) - 0.9 % + 2 4 CaHPO4 4.7 % - MgPO4 - 1.5% CaPO4 7.6 % + + PO3- (free) - 0.01% MgHPO4 4 2- 41.4% NaPO4 - 0.01 % 3- o Calculated speciation of PO4 in seawater of 34.8% at 20 C and a pH OF 8.0 Figure by MIT OCW. P cycling in seawater Dissolved P fractions in are operationally defined: Soluble Reactive Phosphorus (SRP) is measured in filtered seawater as the fraction of phosphate that can be measured directly by the colorimetric phosphomolybdate method. This fraction is believed to consist mainly of inorganic phosphate, but may also encompass some acid- labile organic compounds. Total Dissolved Phosphate (TDP) is measured by the phosphomolybdate method in filtered seawater, after treatment with a strong oxidizing reagent. Soluble Non-Reactive Phosphate (SNP) is calculated by difference (SNP=TDP-SRP) and is thought to consist mainly of dissolved organic phosphate (DOP) and polyphosphates. P cycling in seawater SNP (or DOP) is a large % of total dissolved P in surface oligotrophic waters (Wu et al., 2000) Is biolimiting so most of it is within phytoplankton cells DOP is converted back to inorganic P via enzymatic and microbial remineralization processes Atmosphere 0.9 10-3 Oceanic biota reservoir smaller than 0.03 0.15 < 0.01 Land Biota: 102 Surf. Ocean 33 Ocean Biota: land reservoir BUT the fluxes 0.9 102 32.7 4 2 2 between reactive P & the biota are Land: 6.5 103 0.2 0.3 Deep Ocean much larger 0.4 0.7 3 Minable P 2.8 10 2 3 10 12 12 0.6 0.1 moles/y 4 x 10 moles / 33 x 10 moles yr-1 1.3 108 Crust + Sediment ~ 0.12 years Inventories = 1012 moles (bold) Fluxes = 1012 moles / year Dominance of single celled organisms with short lifetimes Figure by MIT OCW. P cycling in seawater – field methods • Can be studied using 2 natural radioactive P isotopes: 32P (14.3 dys) and 33P (25.3 dys), cosmogenic isotopes from Argon Rapidly removed onto aerosols, added to seawater via rain • 33P/32P in rainwater varies little but 33P/32P ⇑ with time 33 32 (λ33 - λ32)t [ P/ P]o e • High 33P/32P = an older P pool P cycling in seawater – field methods • Measure P isotopic ratio in different pools (inorganic P, DOP, phytoplankton, zooplankton, etc.) Estimate age Turnover of P within the pools Pathways of P through the food chain • Need to constrain the 33P / 32P of rain water • Preliminary studies ⇒ DOP turnover rates vary widely in space and time P cycling in seawater – turnover time Atmosphere 0.9 10-3 Surface seawater P turnover: 12 12 0.03 0.15 < 0.01 90 x 10 moles / 33 x 10 Land Biota: 102 Surf.
Details
-
File Typepdf
-
Upload Time-
-
Content LanguagesEnglish
-
Upload UserAnonymous/Not logged-in
-
File Pages33 Page
-
File Size-