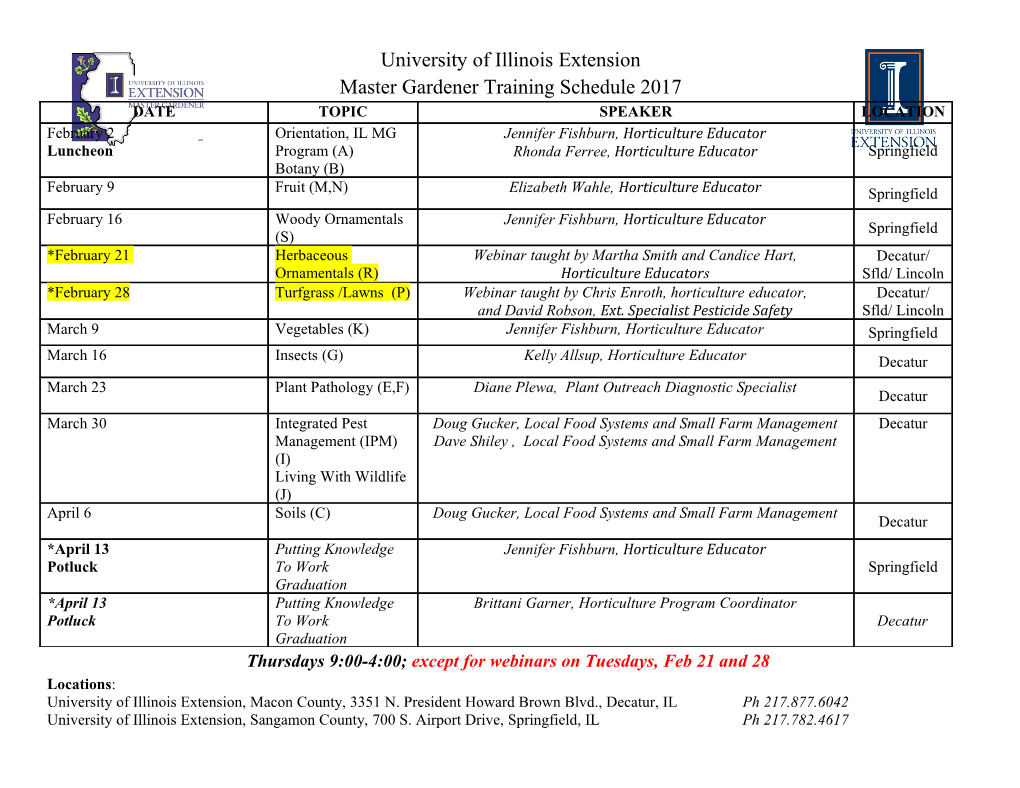
Western Washington University Western CEDAR WWU Honors Program Senior Projects WWU Graduate and Undergraduate Scholarship Spring 2021 Insulin: Roles and Functions in Biochemistry and U.S. Healthcare Mia Brinkley Western Washington University Follow this and additional works at: https://cedar.wwu.edu/wwu_honors Part of the Cell and Developmental Biology Commons, and the Chemistry Commons Recommended Citation Brinkley, Mia, "Insulin: Roles and Functions in Biochemistry and U.S. Healthcare" (2021). WWU Honors Program Senior Projects. 452. https://cedar.wwu.edu/wwu_honors/452 This Project is brought to you for free and open access by the WWU Graduate and Undergraduate Scholarship at Western CEDAR. It has been accepted for inclusion in WWU Honors Program Senior Projects by an authorized administrator of Western CEDAR. For more information, please contact [email protected]. Mia Brinkley Insulin: Roles and Functions in Biochemistry and U.S. Healthcare There is a long list of drugs that treat conditions affecting a large population of the U.S. today. Genetic predisposition, individual lifestyle, and environmental factors all influence many of the most prominent diseases in our population, and the development of drugs as a treatment strategy has brought about both burdens and benefits for the patient. One example of one of these drugs is insulin, with 30% of Diabetes patients using insulin.1 Today, over 8.3 million people are prescribed insulin, and that number is expected to grow substantially over time.1 This massive market is controlled by a small number of manufacturers, causing high cost of insulin and low accessibility for patients. While a higher percentage of the U.S. population has Type 2 Diabetes than Type 1, I will be focusing on Type 1 because of its more limited range of treatment options. Type 1 Diabetes occurs in over 30 million Americans.1 It is caused by an autoimmune process in which CD8 positive cytotoxic T cells recognize beta-cell autoantigens by recognizing MHC class I peptide complexes. This produces a chronic inflammatory response to eliminate insulin-producing beta cells, causing insulin deficiency and hyperglycemia.2 In previous studies, glutamic acid decarboxylase (GAD) and ICA 512 protein tyrosine phosphatase (in 60-85% of patients) have been found to help in elimination of insulin.2 Insulin is naturally produced by the body, secreted by pancreatic beta-cell in the Figure 1. Insulin leaves β-cells of pancreatic Isles of Langerhans Islets of Langerhans as a 51- when stimulated. Pipeline includes the pancreas, an Islet of 3 Langerhans surrounded by acinar cells, and β-cell that is stimulated to residue protein. This release produce and release insulin. Image created using BioRender. is pictured in Figure 1, showing the release of insulin from pancreatic β- cells. The initial protein, proinsulin, has an A and B chain connected by disulfide bonds, as shown in the Figure 2, which is post-translationally modified to form mature insulin.3 Insulin contains three alpha helices: A1- Figure 2. Animation of insulin molecule.3 A8, A12-A18, and B9-B19, containing one intra and two interchain disulfide bonds4 (Figure 3). Insulin works by binding to the insulin receptor (IR), a homodimer with two alpha subunits, each at 135 kDa; two beta subunits, each at 95 kDa; and two minor glycoprotein (gamma) subunits, each at 210 kDa.5 The alpha subunit works to bind insulin, and the beta subunit consists of a tyrosine kinase that phosphorylates itself at a phosphotyrosine, phosphoserine, and phosphothreonine (Figure 4). The gamma subunit is the transmembrane portion, extending through the plasma membrane to connect to the inside of the cell. Figure 3. Structure of insulin molecule. Alpha and beta chains are colored orange and teal, respectively, with intra and inter-disulfide bonds labeled with stars. B A Figure 4. A) Overview of tyrosine kinase subunit of insulin receptor protein. Tyrosine kinase is colored cyan, the swinging loop region is colored magenta, the two Mg2+ coenzymes are colored red, and multicolored is an ATP analogue, with carbon green, nitrogen blue, oxygen red, and sulfur orange. A B Figure 5. Tyrosine kinase undergoes transitional change upon ATP binding. A) Inactivated tyrosine kinase region of insulin receptor protein. Highlighted in magenta is part of the loop that swings during activation, to allow entry into the active site. B) Activated tyrosine kinase, highlighting the loop swing. plasamembrane to connect to the inside of the cell. When insulin binds to one of the receptor’s alpha subunit, it causes a shape change that is propagated into the intracellular region and activates the tyrosine kinases.6 The transition from the inactive to the active state is shown in Figure 5. The active site binds to ATP, which it uses to phophorylate its targets. The transition between the inactive and active state is characterized by several tyrosines being phosphorylated, causing a loop portion to swing out of the active site and allow ATP and magnesium entry for enzymatic process.6 All of this information that’s known about the insulin protein and insulin receptor is helpful to researchers to maximize efficiency of Diabetes drugs today, but this information has not been known nearly as long as Diabetes has been around. Treatments to Type 1 Diabetes, prior to the use of insulin as a drug, were trying and physically exhausting. Developed in the early 20th century, starvation therapy was a way to keep sugar below tolerance levels, measured routinely in urine. This would hopefully prevent organ failure, but it meant that a patient had to restrict themselves to 800 calories per day.7 This caused weakness and low resistance to infections, as well as a generally decreased quality of life. Without treatment, diabetes would start with weight loss, listlessness, hunger, and thirst, as ketones clog organs and the kidneys begin to fail. Eventually, coma and then death would ensue. Treatments were becoming more and more important as the 20th century brought cleaner water and better sewage. As child mortality rates declines, the rates of Type 1 Diabetes increased with the growing population. Type 2 also increased, as being larger was a sign of wealth and prosperity. A better treatment was imperative. However, it wasn’t for lack of trying that the treatment development was slow. Since 1869, when Paul Langerhans identified insulin-secreting pancreatic cells that floated in clusters of acinar cells—these clusters called islets of Langerhans—there was work on cats, dogs, guinea pigs, and rabbits to find pancreatic extract for treating patients.7 One group in 1912 thought that bacterium in milk would cleanse the alimentary canal from excess sugar. Patients were, of course, told to drink more milk, not knowing of the sugar contained in what they were drinking. In 1915, J.D. Rockefeller founded a medical research institute, let by Israel Kleiner, to work on pancreatic extracts. Kleiner’s method was to grind up dog pancreases, put them in solution with salt, and inject them into the now-diabetic dogs. He found 50% decline in blood sugar levels, but the negative side effects prevented further investigation.7 The world-changing science was in 1914, when a group of scientists at University of Toronto banded together to find a way to extract insulin (Figure 6). The team was led by J.J.R. Macleod and included F.G. Banting, C.H. Best, J.B. Collin, J.Hepburn, J.K. Latchford, and E. Clark Noble. They used rabbits and developed manufactured pancreatic extract, first calling it “isletin.” At first in an oral form— given to Banting’s friend Dr. Joseph Gilchrist—with no beneficial results, it was Figure 6. Cover of Toronto Daily Star, published on March 22, 1922, showing the four doctors leading the insulin treatment research. later successfully given as an injection to Leonard Thompson, a 14-year old patient of Toronto General Hospital.8 The Toronto group, on January 25, 1922, settled an agreement with Connaught Anti-Toxin Laboratories for the manufacturing of this extract, but the production failed by March. Collin and Best succeeded in regaining the formula in May. By May 3rd, the extract was successfully extracted from cow pancreas and was first referred to as insulin by Macleod. Elizabeth Hughes Gossett, daughter of U.S. statesman Charles Evans Hughes, was the famous recipient of the first insulin treatment for Type 1 diabetes, receiving over 42,000 shots from age 12 until her death.7 Banting refused to put the patent for insulin in his name, believing it would be unethical for a doctor to benefit from the discovery of a life-saving treatment; Collin and Best respected this decision and agreed to sell the patent for insulin for $1 to the University of Toronto. The intention was to provide the quickest availability to the public and allow anyone to prepare the extract with no profitable monopoly to develop.9 While this was meant to prevent monopolizing on the drug production and distribution, it did little in the end for the benefits of patients. The University formed the Diabetic Clinic in Toronto General Hospital in June of 1922 and established the Insulin Committee in August to control licensing, patenting, and trademarking of the new drug.8 The teamed with Eli Lilly to allow it to take out any U.S. patents “for manufacturing improvements, but the university would receive the patent right for the rest of the world, and they let other companies around the world receive the patent from the University.9 By May of 1923, insulin was made commercially available in Great Britain, and by June, the initial agreement with Eli Lilly ended, allowing other pharmaceutical companies to apply for licenses for distribution. By October of 1923, insulin was made commercially available in the U.S.
Details
-
File Typepdf
-
Upload Time-
-
Content LanguagesEnglish
-
Upload UserAnonymous/Not logged-in
-
File Pages20 Page
-
File Size-