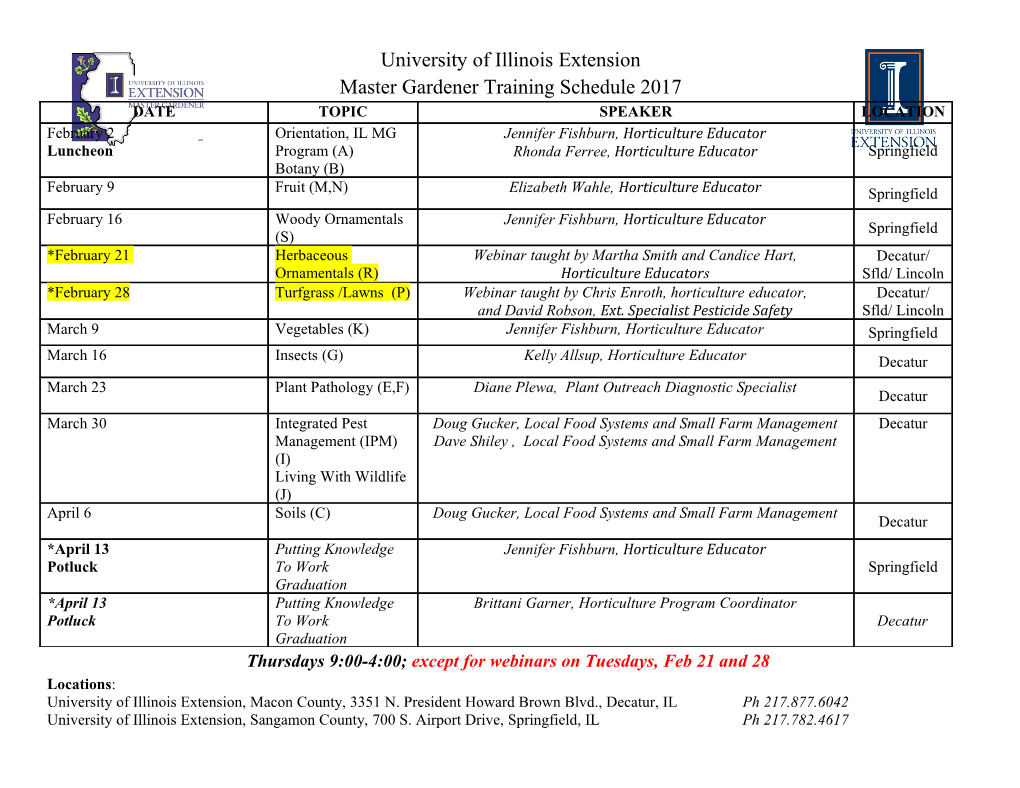
Acoustically trapped colloidal crystals that are reconfigurable in real time Mihai Caleap1 and Bruce W. Drinkwater Faculty of Engineering, University of Bristol, Bristol BS8 1TR, United Kingdom Edited* by John B. Pendry, Imperial College London, London, United Kingdom, and approved March 5, 2014 (received for review December 13, 2013) Photonic and phononic crystals are metamaterials with repeating tuned, they do not facilitate real-time reconfigurability and so unit cells that result in internal resonances leading to a range of cannot be used in an active mode. wave guiding and filtering properties and are opening up new In this article we report for the first time, to our knowledge, applications such as hyperlenses and superabsorbers. Here we the experimental realization of a 3D colloidal metamaterial that show the first, to our knowledge, 3D colloidal phononic crystal is reconfigurable in real time and demonstrate its ability to that is reconfigurable in real time and demonstrate its ability to rapidly alter its acoustic filtering characteristics. The reconfig- rapidly alter its frequency filtering characteristics. Our reconfig- urable metamaterial is assembled from a low-density aqueous urable material is assembled from microspheres in aqueous solu- suspension of microspheres. Our metadevice generates a high- tion, trapped with acoustic radiation forces. The acoustic radiation amplitude megahertz-frequency (MHz) acoustic standing wave force is governed by an energy landscape, determined by an which results in acoustic radiation forces that lead to particles applied high-amplitude acoustic standing wave field, in which moving to, and becoming trapped in, a simple orthorhombic particles move swiftly to energy minima. This creates a colloidal lattice (although here we explore only simple tetragonal forms). crystal of several milliliters in volume with spheres arranged in an By varying the frequency of the acoustic standing wave in the orthorhombic lattice in which the acoustic wavelength is used to metadevice we are able to control the lattice geometry. We use control the lattice spacing. Transmission acoustic spectroscopy transmission acoustic spectroscopy to explore the reconfigur- shows that the new colloidal crystal behaves as a phononic meta- ability of the resulting metamaterial. These measurements reveal material and exhibits clear band-pass and band-stop frequencies a complex and tunable distribution of band-gap and band-stop ENGINEERING which are adjusted in real time. phenomena over a wide range of frequencies. reconfigurable acoustic assembly | acoustic metamaterials | Results aqueous suspension of microspheres The metadevice used to form the 3D reconfigurable meta- material is summarized in Fig. 1. To generate the desired lattice rtificially engineered metamaterials have attracted signifi- pattern, the metadevice creates a high-amplitude acoustic Acant research interest due to their useful wave guiding and standing wave field using five planar piezoceramic transducers transmission properties. The interest in these materials stems from arranged as shown in Fig. 1A. The metadevice consists of three the possibility of gaining previously unheralded control over wave orthogonally arranged systems: the levitation system, arranged phenomena, for example, controlling the path of waves leads to vertically, which lifts the particles and holds them in horizontal incredibly efficient lenses (1) or invisibility cloaking (2, 3) and planes, and two orthogonal manipulation stages, which use the controlling their transmission and reflection leads to highly ef- counterpropagating wave method to produce a grid of acoustic ficient filters (4), diodes (5–7), or superabsorbers (8). traps in the horizontal plane. The lattice spacing can be con- Photonic and phononic crystals are periodic metamaterials trolled by the frequencies of the standing waves and the form typically created from multiple elementary repeating cells. They and location of the lattice by the phase differences (16). The exhibit a well-known series of band-pass and band-stop fre- quencies that have attracted significant attention for use as filters Significance and absorbers. Here we demonstrate a phononic crystal recon- figurable in real time, although the same principles could be used We have been working on metamaterials that are reconfig- to fabricate other metamaterials including photonic crystals, as urable in real time with a view to creating genuinely active well as the more elaborate configurations required for applica- metamaterials. Such materials will allow researchers to gain tions such as cloaking. unprecedented control over a range of optical and acoustic The frequencies of the band gaps in phononic crystals can be wave phenomena. To date, although numerous examples of tuned by changing the lattice geometry (9), and the width of the metamaterials exist, none is reconfigurable in three dimensions. gaps depends on the contrast between the densities and sound We have developed a method for creating three-dimensional velocities of the component materials. Most of the work to date colloidal crystals that are reconfigurable in real time. Our method has not involved any reconfigurability, for example, 2D phononic uses acoustic assembly to trap a suspension of microspheres in crystals with a periodicity millimeter order and above have been patterns resembling crystal lattices. We show that it is possible to assembled manually and used to explore their basic properties dynamically alter the geometry of the colloidal crystal and use (10, 11). A reconfigurable phononic crystal has been created in our metamaterial as an ultrasonic filter that can be tuned in 2D using optical tweezers but this is limited to a relatively small real time. scale (’ 1 × 108-m2 area) and to transparent or dielectric particles (12). Three-dimensional experimental realizations of phononic Author contributions: M.C. and B.W.D. designed research; M.C. performed research; M.C. crystals have either been manufactured with millimeter period- and B.W.D. analyzed data; and M.C. and B.W.D. wrote the paper. icity or as colloids (13, 14). Colloids represent a particularly at- The authors declare no conflict of interest. tractive option as they are known to self-assemble into simple 3D *This Direct Submission article had a prearranged editor. crystalline structures. Recent work on 3D hypersonic (GHz) col- Freely available online through the PNAS open access option. loidal crystals has led to experimental observation of the hyper- 1To whom correspondence should be addressed. E-mail: [email protected]. sonic Bragg gaps (15). However, whereas the choice of particle This article contains supporting information online at www.pnas.org/lookup/suppl/doi:10. shape, size, volume fraction, etc. allows the colloidal crystal to be 1073/pnas.1323048111/-/DCSupplemental. www.pnas.org/cgi/doi/10.1073/pnas.1323048111 PNAS Early Edition | 1of5 Downloaded by guest on September 27, 2021 Fig. 1. Acoustic metadevice capable of manipulat- ing the acoustic space and controlling the propa- gation of waves. (A) Schematic view of the fabri- cated metadevice with piezoceramic transducers for generating standing acoustic waves and a pair of broadband transducers for acoustic transmission spectroscopy. Note a photograph of the device can be seen in SI Text, Fig. S4.(B) Acoustic manipulator with four transducers, positioned as two orthogonal pairs. Exciting weakly resonant waves in the piezo- ceramic plates, polystyrene microspheres suspended in a fluid are driven to the pressure nodes of the standing wave field. (C) Acoustic levitator with one transducer at the base of the device holds particles against gravity in horizontal planes inside a thin- wall transparent polyester tube; the free surface of the aqueous suspension above the levitator acts as a reflector to form standing waves. (D) Experimental apparatus for acoustic transmission spectroscopy. The ultrasonic pulse generated by a monolithic transducer is incident upon the colloidal crystal in the center of the device and the transmitted signal is collected by a 2D matrix array transducer whose center elements are collinear with the incident wave. The image in the background shows a typical acoustic scattering be- havior of a colloidal crystal lattice at an instant time. Arrows indicate direction of propagation of the waves. resultant 3D standing wave exerts an acoustic radiation force on cited in-phase, the particles were further formed into ap gridffiffiffi in the particles (17). This force stems from a combination of the the horizontalpffiffiffi X À Y plane with lattice spacing ax = λx= 2 and time-averaged acoustic pressure and inertial interaction between ay = λy= 2. Here the wavelengths in the X- and Y directions, λx the particles and the acoustic field (18). Under Rayleigh scat- and λy, were used to reconfigure the metamaterial (see the sec- tering conditions, the wavelength is larger than the particle size ond section of SI Text). Typical acoustic landscape is depicted ðλ >> aÞ and acoustic radiation force due to a standing wave is in Fig. S2, while the particle positions are shown in Fig. S3. governed by the spatial gradient of the energy landscape, U, i.e., The acoustic standing wave patterns were first characterized F = −∇U (17). Particles with densities greater than the fluid ex- by a Schlieren optical system which maps the time-averaged perience forces toward pressure nodes. However, whereas this intensity field distribution. Fig. 2 shows the intensity distri-
Details
-
File Typepdf
-
Upload Time-
-
Content LanguagesEnglish
-
Upload UserAnonymous/Not logged-in
-
File Pages5 Page
-
File Size-