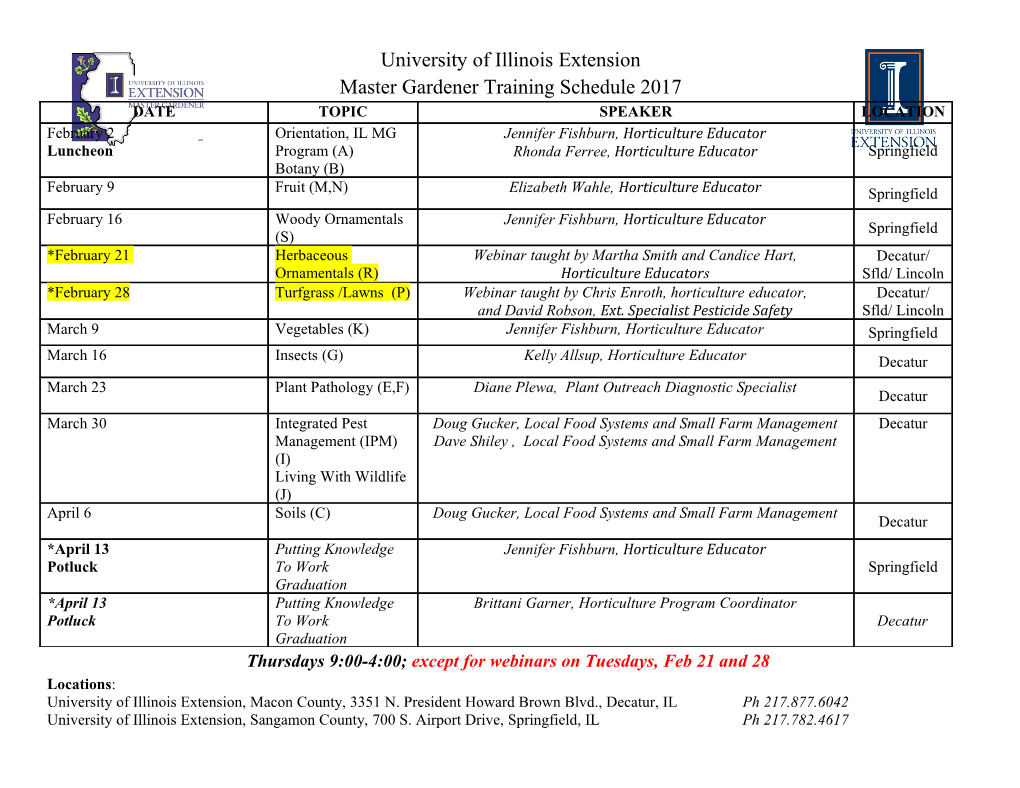
The Pennsylvania State University The Graduate School College of Medicine CHARACTERIZATION OF UGTS ACTIVE AGAINST SUBEROYLANILIDE HYDROXAMIC ACID (SAHA): VARIATIONS IN SAHA GLUCURONIDATION ASSOCIATED WITH UGT GENETIC VARIANTS A Dissertation in Genetics by Renee M. Balliet © 2009 Renee M. Balliet Submitted in Partial Fulfillment of the Requirements for the Degree of Doctor of Philosophy August 2009 The dissertation of Renee M. Balliet was reviewed and approved* by the following: Philip Lazarus Professor of Pharmacology Associate Director of the Cancer Institute Dissertation Adviser Chair of Committee Laura Carrel Assistant Professor of Biochemistry and Molecular Biology Lisa M. Shantz Associate Professor of Cellular and Molecular Physiology Christopher Herzog Associate Professor of Pharmacology Gavin Robertson Co-Chair of Genetics Department *Signatures are on file in the Graduate School. ii ABSTRACT Suberoylanilide Hydroxamic Acid (SAHA) is a histone deacetylase inhibitor used in the treatment of cutaneous T-cell lymphoma and in clinical trials for treatment of multiple other cancers. Variations in patient response and toxicities have been observed; however, no underlying cause has been identified for these differences. A mechanism that could be responsible for the observed differences is altered drug metabolism. A major mode of SAHA metabolism is by glucuronidation via the UDP- glucuronosyltransferase (UGT) family of enzymes. The UGT superfamily of enzymes catalyzes the glucuronidation of a variety of endogenous compounds such as bilirubin and steroid hormones, as well as xenobiotics such as drugs and environmental carcinogens. These enzymes are located in the endoplasmic reticulum of cells and make xenobiotics and endogenous compounds more water soluble through their conjugation to glucuronic acid in a reaction with the hydrophilic co-substrate, UDPGA. This conjugation alters the biological properties of the compound to enhance its excretion in the urine or bile and typically converts substrates into products that are less pharmacologically active. These enzymes have been associated with altered drug metabolism and correlated with patient response and toxicity. The primary goals of this thesis include: identification of the UGTs responsible for SAHA glucuronidation, characterization of the UGTs that are most active against SAHA, in vitro studies of UGT genotype/SAHA glucuronidation phenotype association, and characterization of the promoter region of the UGT1A10 gene. To identify and characterize the UGTs active against SAHA, homogenates from UGT-overexpressing HEK293 cell lines were used. The hepatic UGTs 2B17 and 1A9 and the extra-hepatic UGTs 1A8 and 1A10 exhibited the highest overall activity against SAHA as determined . -1. -1 by Vmax/KM (16±6.5, 7.1±2.2, 33±6.3, and 24±2.4 nL min mg UGT protein , respectively), with UGT2B17 exhibiting the lowest KM (300 µM) for SAHA of any UGT in vitro. While the UGT1A8p.Ala173Gly variant exhibited a 3-fold (P<0.005) decrease in glucuronidation activity for SAHA as compared to wild-type UGT1A8, the UGT1A8p.Cys277Tyr variant exhibited no detectable glucuronidation activity; a similar iii lack of detectable glucuronidation activity was observed for the UGT1A10p.Gly139Lys variant. To analyze the effects of the UGT2B17 gene deletion variant (UGT2B17*2) on SAHA glucuronidation phenotype, human liver microsomes (HLM) were analyzed for glucuronidation activity against SAHA as well as for UGT2B17 genotype. HLM from subjects homozygous for UGT2B17*2 exhibited a 45% (P<0.01) decrease in SAHA glucuronidation activity and a 75% (P<0.002) increase in KM for SAHA as compared to the HLMs from subjects homozygous for the wild-type UGT2B17*1 allele. Further stratification of the HLM glucuronidation data by gender, identified a significant difference in SAHA glucuronidation between males and females, with females having significantly less glucuronidation. Characterization of the promoter of one of the most active glucuronidators of SAHA, UGT1A10, led to the identification of a novel deletion which was able to cause differential induction of luciferase reporter activity in colon derived cell lines. These data suggested that tissue specific regulation of UGTs may ultimately determine glucuronidation levels in specific tissues. Overall, these results suggest that several UGTs play important roles in the metabolism of SAHA and that females and UGT2B17-null males could potentially exhibit altered SAHA clearance rates and differences in overall response to the drug. iv TABLE OF CONTENTS List of Figures ix List of Tables xii List of Abbreviations xiii Acknowledgements xv Chapter 1: Literature Review 1 1.1 Introduction 1 1.1.1 Individualized Medicine and Genetic Variation 6 1.1.2 Cancer as a Complex Disease 4 1.2 HATs and HDACs 10 1.2.1 HATs 12 1.2.2 HDACs 13 1.2.3 HDAC Inhibitors 16 1.2.4 SAHA 19 1.2.5 SAHA Metabolism 24 1.3 UDP-glucuronosyltransferase 27 1.3.1 Gene Structure 29 1.3.2 Expression and Regulation 41 1.3.3 Endogenous Substrates 42 1.3.4 Exogenous Substrates 42 1.3.5 Genetic Variants 43 1.3.6 Pharmacogenetics and UGTs 45 Chapter 2: Identification and Characterization of UGTs against SAHA 51 2.1 Introduction 51 2.2 Materials and Methods 54 2.2.1 Generation of UGT-overexpressing cell lines 54 2.2.2 Preparation of cell homogenates 60 2.2.3 Glucuronidation Assays 61 2.2.4 SAHA-glucuronide confirmation 63 2.2.5 Kinetic analysis of interactions of UGTs with SAHA 64 v 2.3 Results 65 2.3.1 Characterization of UGT-overexpressing cell lines 65 2.3.2 Confirmation of SAHA-glucuronide formation 67 2.3.3 Screen of individual UGTs for activity against SAHA 71 2.3.4 Kinetic parameters for the glucuronidation of SAHA by individual UGT family members 75 2.4 Summary 79 Chapter 3: Characterization of the ability of variant UGTs to glucuronidate SAHA 81 3.1 Introduction 81 3.2 Materials and Methods 84 3.2.1 Generation of cell lines overexpressing UGT variants 84 3.2.2 Preparation of cell homogenates 86 3.2.3 Glucuronidation assays 87 3.2.4 Kinetic analysis of variant UGTs against SAHA 89 3.3 Results 90 3.3.1 Characterization of HEK293 cell lines overexpressing UGT variants 3.3.2 Screen variant UGTs for activity against SAHA 90 3.3.3 Results of screening for glucuronidation of SAHA by UGT variants 96 3.4 Summary 99 Chapter 4: Characterization of SAHA glucuronidation by UGT in human tissue samples 103 4.1 Introduction 103 4.2 Materials and Methods 106 4.2.1 Human Liver Tissue 106 4.2.2 Preparation of Human Liver Microsomes 106 4.2.3 Isolation of DNA and RNA from tissue used to prepare HLM 107 4.2.4 Reverse transcription of RNA 109 4.2.5 Genotyping Assay for UGT2B17 109 4.2.5.1 Multiplex Real-Time PCR assay to determine UGT2B17 genotype 110 4.2.5.2 Copy Number Variation 111 vi 4.2.6 Gender Identification Assay 112 4.2.7 Glucuronidation Assays for SAHA in HLM and Colon homogenates 106 4.2.8 Expression Assay for UGT2B17 113 4.3 Results 115 4.3.1 Analysis of UGT2B17 Genotype 115 4.3.2 UGT2B17 expression in HLM 120 4.3.3 UGT2B17 genotype/phenotype correlation in HLM 123 4.3.4 Confirmation of the gender of each HLM preparation and stratification of UGT2B17 expression and glucuronidation results by gender. 127 4.3.5 SAHA activity in colon homogenate 131 4.4 Summary 133 Chapter 5: UGT1A10 Promoter Study 138 5.1 Introduction 138 5.2 Materials and Methods 143 5.2.1 Human DNA used to screen for UGT1A10 polymorphisms 143 5.2.2 Amplification and Sequencing of the UGT1A10 promoter 143 5.2.3 Real-Time PCR genotype assay for novel UGT1A10 promoter deletion 144 5.2.4 Cloning of UGT1A10 promoter constructs into luciferase vector 145 5.2.5 Luciferase Assay for UGT1A10 constructs 148 5.2.6 Induction of Luciferase via UGT1A10 promoter 149 5.3 Results 151 5.3.1 Confirmation of Amplification and Sequencing of the UGT1A10 promoter 151 5.3.2 Genotypes observed for the UGT1A10 promoter 152 5.3.3 Effects of the UGT1A10 promoter region and its variants on gene expression 153 5.3.4 Effect of treatment with hypothesized inducers on UGT1A10 promoter driven luciferase activity 158 5.4 Summary 159 Chapter 6: General Discussion and Future Directions 163 vii Appendix A. Nucleotide Sugar Transporters 178 A.1 Introduction 178 A.2 Materials and Methods 182 A.2.1 Identification of SNPs 182 A.2.2 DNA Samples 182 A.2.3 Multiplex PCR assay 182 A.2.4 SNaPshot Assay for SNPs 185 A.3 Results 188 A.3.1 Identification of SNPs 188 A.3.2 Development of Multiplex PCR Assay to Analyze Nonsynonymous 186 SNPs of the NSTs 6 A.3.3 Optimization of SNaPshot Assay for SNPs 193 A.4 Summary 198 REFERENCES 202 viii LIST OF FIGURES Figure Page 1.1 Schematic of the structure of histones in nucleosomes 11 1.2 Many functions of HDACs 15 1.3 Structure of trichostatin A and SAHA 21 SAHA inhibits HDAC activity by binding to the pocket of the catalytic 1.4 21 site 1.5 Schematic of SAHA metabolism 25 Phylogenetic tree showing the relationships between the human UDP- 1.6 30 glucuronosyltransferases (UGTs) Genomic organization of the UGT1A locus and example of mRNA 1.7 31 transcribed 1.8 The UGT2B family locus 32 1.9 Putative protein structure of UGT proteins 33 1.10 Comparison of the UGT1 locus in human, rat, and mouse 35 1.11 Expression patterns of UGTs in human tissue 37 Schematic representation of the UGT1 gene and the alternative 1.12 41 splicing events taking place at the 5' and 3' ends of the locus The effect of the mdr-1 SNP G2677T/A on the paclitaxel treatment 1.13 46 outcome Schematic diagram of the pcDNA3.1/V5-His-TOPO mammalian 2.1 57 expression vector Western blot analysis
Details
-
File Typepdf
-
Upload Time-
-
Content LanguagesEnglish
-
Upload UserAnonymous/Not logged-in
-
File Pages242 Page
-
File Size-