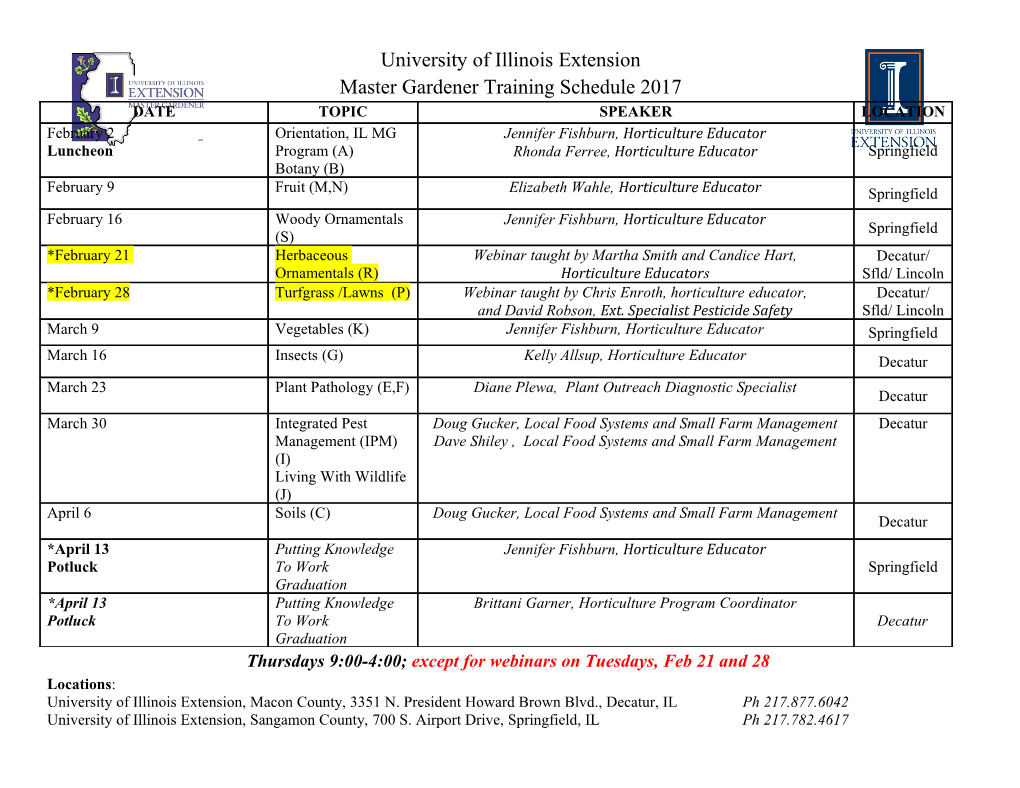
Atmos. Chem. Phys., 18, 13013–13030, 2018 https://doi.org/10.5194/acp-18-13013-2018 © Author(s) 2018. This work is distributed under the Creative Commons Attribution 4.0 License. Chlorine oxidation of VOCs at a semi-rural site in Beijing: significant chlorine liberation from ClNO2 and subsequent gas- and particle-phase Cl–VOC production Michael Le Breton1, Åsa M. Hallquist2, Ravi Kant Pathak1, David Simpson3,4, Yujue Wang5, John Johansson3, Jing Zheng5, Yudong Yang5, Dongjie Shang5, Haichao Wang5, Qianyun Liu6, Chak Chan7, Tao Wang8, Thomas J. Bannan9, Michael Priestley9, Carl J. Percival9,a, Dudley E. Shallcross10,11, Keding Lu5, Song Guo5, Min Hu5, and Mattias Hallquist1 1Department of Chemistry and Molecular Biology, University of Gothenburg, Gothenburg, Sweden 2IVL Swedish Environmental Research Institute, Gothenburg, Sweden 3Earth and Space Sciences, Chalmers University of Technology, Gothenburg, Sweden 4Norwegian Meteorological Institute, Oslo, Norway 5State Key Joint Laboratory of Environmental Simulation and Pollution Control, College of Environmental Sciences and Engineering, Peking University, Beijing, China 6Division of Environment and Sustainability, Hong Kong University of Science and Technology, Clearwater Bay, Kowloon, Hong Kong 7School of Energy and Environment, City University of Hong Kong, Hong Kong 8Department of Civil and Environmental Engineering, Hong Kong Polytechnic University, Hong Kong 9Centre for Atmospheric Science, School of Earth, Atmospheric and Environmental Science, University of Manchester, Manchester, UK 10School of Chemistry, University of Bristol, Cantock’s Close, Bristol, UK 11Department of Chemistry, University of the Western Cape, Bellville, Cape Town, South Africa anow at: Jet Propulsion Laboratory, California Institute of Technology, 4800 Oak Grove Drive, Pasadena, CA, USA Correspondence: Michael Le Breton ([email protected]) Received: 9 January 2018 – Discussion started: 16 January 2018 Revised: 4 August 2018 – Accepted: 21 August 2018 – Published: 11 September 2018 Abstract. Nitryl chloride (ClNO2) accumulation at night acts CO and benzene, which often persisted at high levels after as a significant reservoir for active chlorine and impacts the sunrise until midday. This was attributed to such high mix- following day’s photochemistry when the chlorine atom is ing ratios persisting after numerous e-folding times of the liberated at sunrise. Here, we report simultaneous measure- photolytic lifetime enabling the chlorine atom production to 5 −3 ments of N2O5 and a suite of inorganic halogens including reach 2.3 × 10 molecules cm from ClNO2 alone, peaking 5 −3 ClNO2 and reactions of chloride with volatile organic com- at 09:30 LT and up to 8.4 × 10 molecules cm when in- pounds (Cl–VOCs) in the gas and particle phases utilising the cluding the supporting inorganic halogen measurements. Filter Inlet for Gas and AEROsols time-of-flight chemical Cl–VOCs were observed in the particle and gas phases ionisation mass spectrometer (FIGAERO-ToF-CIMS) dur- for the first time at high time resolution and illustrate how ing an intensive measurement campaign 40 km northwest the iodide ToF-CIMS can detect unique markers of chlorine of Beijing in May and June 2016. A maximum mixing ra- atom chemistry in ambient air from both biogenic and an- tio of 2900 ppt of ClNO2 was observed with a mean cam- thropogenic sources. Their presence and abundance can be paign nighttime mixing ratio of 487 ppt, appearing to have explained via time series of their measured and steady-state an anthropogenic source supported by correlation with SO2, calculated precursors, enabling the assessment of competing Published by Copernicus Publications on behalf of the European Geosciences Union. 13014 M. Le Breton et al.: Chlorine oxidation of VOCs at a semi-rural site in Beijing OH and chlorine atom oxidation via measurements of prod- cal abundance (ROx D OH C HO2 C RO2), O3 produc- ucts from both of these mechanisms and their relative contri- tion rate, NOx lifetime and partitioning between reactive bution to secondary organic aerosol (SOA) formation. forms of nitrogen (Riedel et al., 2014). The rate constants for the reaction of chlorine atoms with a number of VOCs is around 200 times larger than the equivalent reaction with OH 1 Introduction (Tanaka et al., 2003); therefore, its abundance, fate and cy- cling can significantly alter standard daytime oxidation path- ways. The oxidation of VOCs by chlorine atoms is thought to NO and NO2 (NOx) are important catalysts in the photo- be significant in the early hours of the day while OH mixing chemical production of ozone (O3) playing a significant role in the oxidation of volatile organic compounds (VOCs) and ratios are low and chlorine atom production is high through subsequently have an adverse effect on air quality. In the day- the photolysis of ClNO2, as well as feeding into the standard HO = NO cycles via production of peroxy radicals from re- time, NOx is primarily removed by the hydroxyl radical (OH) x x actions with alkanes. Additional Cl photolysis and HCl re- to form nitric acid (HNO3), which is subsequently lost by 2 wet deposition, becoming a major component of acid rain. action with OH can also produce chlorine atoms throughout At night, the OH radical is not a significant oxidant as pho- the day but at lower rates. Saturated hydrocarbons are usually oxidised by reaction tolysis stops, enabling the reaction between NO2 and O3 to with OH or a chlorine atom to form an organic peroxy rad- form significant levels of the nitrate radical (NO3) (Atkin- ical (RO ), and H O or HCl depending on the oxidant (Re- son, 2000). NO3 can accumulate at night or further react with 2 2 actions R12 and R13), which is the dominant pathway for NO2, leading to the formation of N2O5 (Brown et al., 2003; Brown and Stutz, 2012). This equilibrium can lead to the re- chloride–VOC (Cl–VOC) reactions. In a heavily polluted en- vironment such as Beijing, the RO favours further reactions action of NO3 with VOCs at night forming organic nitrates or 2 act as an important intermediate for heterogeneous reaction with NO to form an oxygenated volatile organic compound − C (HO2 and NO2) or an alkyl nitrate (RONO2). Specifically, on aerosols as N2O5 produces NO3 and NO2 in the aque- ous phase (Hallquist et al., 1999, 2000; Wagner et al., 2013). acyl peroxy radicals can also react with NO2 to form acyl In the presence of chlorine, which is assumed in models to peroxy nitrates (APNs) such as peroxy acetyl nitrate (PAN). predominantly come from sea salt (Baker et al., 2016), nit- O2 ryl chloride (ClNO2) can be formed and released into the gas RH C OH −! RO2 C H2O (R12) phase from the aerosol surface (Osthoff et al., 2008). ClNO2 O2 formation thereafter acts as a nighttime radical reservoir due RH C Cl −! RO2 C HCl (R13) to its stability at night. RO2 C NO ! OVOC C HO2 C NO2 (R14a) At sunrise, ClNO2 is rapidly photolysed, liberating the highly reactive chlorine atom subsequently converting it into RO2 C NO ! RONO2 (R14b) ClO, HOCl and ClONO2 depending on the available sun- C $ light, O3, HOx and NOx levels via the following reaction RO2 NO2 APN (R15) pathways (R1–R11). Addition of the chlorine atom to an unsaturated VOC can ClNO2 C hν ! Cl C NO2 (R1) also occur and then continue on the similar reaction path- Cl C O3 ! ClO C O2 (R2) way as denoted by Reactions (R12)–(R15). These pathways result in the production of unique chlorine atom chemistry ClO C NO2 ! ClONO2 (R3) markers which have been previously investigated to indi- C ! C ClO HO2 HOCl O2 (R4) cate the extent of chlorine atom oxidation reactions (Riemer ClONO2 C hν ! Cl C NO3 (R5) et al., 2008; Keil and Shepson, 2006). The utilisation of ClONO2 C hν ! ClO C NO2 (R6) these compounds, such as 2-chloro-peroxypropionyl nitrate HOCl C hν ! Cl C OH (R7) (2-Cl PPN) and 1-chloro-3-methyl-3-butene-2-one (CMBO), C − as chlorine atom chemistry markers relies on the abundance ClONO2 C H C Cl ! Cl2 C HNO3 (R8) of the chlorine atom, the VOC precursor, HOx, NOx and O3 C − HOCl C H C Cl ! Cl2 C H2O (R9) and competing pathways for chlorine atom reactions. Riedel ClO C NO ! Cl C NO2 (R10) et al. (2014) calculated that up to 10 parts per trillion (ppt) Cl–VOCs are formed as a result of chlorine atom addition to OH C HCl ! Cl C H2O (R11) alkenes and can therefore provide a number of potential pe- The liberated chlorine will predominantly react with riods of dominating active Cl chemistry (Wang et al., 2001). VOCs, with the pathways listed (Reactions R2–R11) rep- The production of chloroperoxy radicals via chlorine atom resenting alternative routes to loss of the chloride radical, addition can lead to the formation of semivolatile oxidation and contribute to daytime photochemical oxidation, compet- products which have been observed for both biogenic (Cai ing with OH and perturbing standard organic peroxy radi- and Griffin et al., 2008) and anthropogenic emissions (Huang Atmos. Chem. Phys., 18, 13013–13030, 2018 www.atmos-chem-phys.net/18/13013/2018/ M. Le Breton et al.: Chlorine oxidation of VOCs at a semi-rural site in Beijing 13015 et al., 2014; Riva et al., 2015) in controlled laboratory stud- to a compound that is much more abundant via multi-peak ies. Chlorine-initiated oxidation of isoprene could also rep- fitting. This technique has previously been applied for the resent a significant oxidation pathway due to its rapid reac- measurement of ClNO2 and Cl2 (Faxon et al., 2015) and re- tion rate compared with OH (Orlando et al., 2003) result- cently for Cl–VOCs (D. Wang et al., 2017) in the gas phase. ing in gas-phase products such as chloroacetaldehyde and In this study, a ToF-CIMS utilising the Filter Inlet for Gas CMBO, a unique tracer for atmospheric chlorine atom chem- and AEROsols (FIGAERO) is deployed at a site in semi- istry (Nordmeyer et al., 1997).
Details
-
File Typepdf
-
Upload Time-
-
Content LanguagesEnglish
-
Upload UserAnonymous/Not logged-in
-
File Pages18 Page
-
File Size-