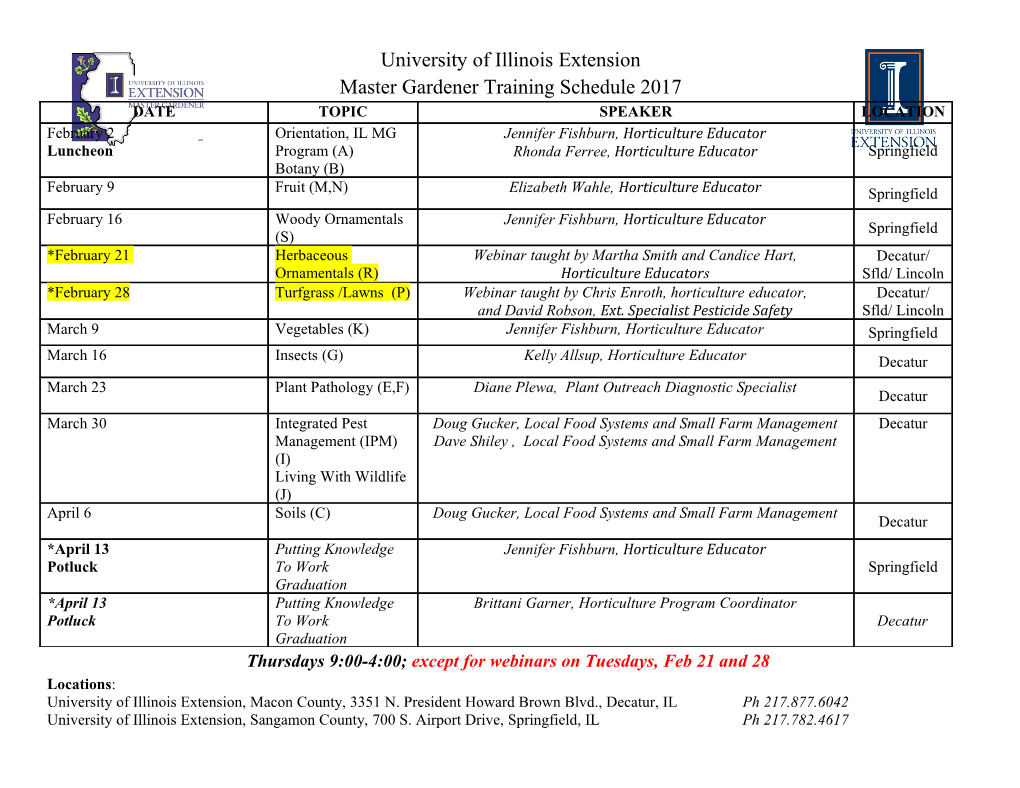
Weak average liquid-cloud-water response to anthropogenic aerosols Article Accepted Version Toll, V., Christensen, M., Quaas, J. and Bellouin, N. (2019) Weak average liquid-cloud-water response to anthropogenic aerosols. Nature, 572 (7767). pp. 51-55. ISSN 0028-0836 doi: https://doi.org/10.1038/s41586-019-1423-9 Available at http://centaur.reading.ac.uk/85364/ It is advisable to refer to the publisher’s version if you intend to cite from the work. See Guidance on citing . To link to this article DOI: http://dx.doi.org/10.1038/s41586-019-1423-9 Publisher: Nature Publishing Group All outputs in CentAUR are protected by Intellectual Property Rights law, including copyright law. Copyright and IPR is retained by the creators or other copyright holders. Terms and conditions for use of this material are defined in the End User Agreement . www.reading.ac.uk/centaur CentAUR Central Archive at the University of Reading Reading’s research outputs online 1 Weak average liquid cloud water response to anthropogenic aerosols Authors: Velle Tolla,b*, Matthew Christensenc, Johannes Quaasd and Nicolas Bellouina a Department of Meteorology, University of Reading, UK b Institute of Physics, University of Tartu, Estonia c Atmospheric, Oceanic and Planetary Physics, University of Oxford, UK d Institute for Meteorology, Universität Leipzig, Germany *Corresponding author: [email protected] Abstract The cooling of the Earth’s climate by anthropogenic aerosols offsets an unknown fraction of greenhouse gas warming. A potentially strong aerosol cooling effect is worrying because it implies a large sensitivity of the Earth’s surface temperature to anthropogenic forcing. Aerosol-induced increases in cloud water have been postulated to lead to a substantial cooling effect. Here we provide direct observational evidence for a relatively weak average decrease in the amount of water in liquid-phase clouds. Polluted clouds downwind of various anthropogenic pollution sources, like oil refineries, smelters, coal-fired power plants, cities, wildfires, and ships, reveal cancellations between aerosol-induced cloud water increases and decreases. We estimate that the decrease in cloud water offsets 23% of the global climate cooling effect caused by aerosol-induced increases in cloud droplet number. These findings invalidate the hypothesis of a substantial climate cooling effect through cloud water increases and translate into reduced uncertainty in the projections of future climate. 2 Main Atmospheric aerosols, tiny solid and liquid particles suspended in the air, affect the climate by direct interaction with radiation and through modulation of cloud properties. Through these effects, anthropogenic aerosols cause an imbalance in the Earth’s radiative budget, called radiative forcing. Aerosols mask an unknown fraction of greenhouse gas radiative forcing and thus cool the climate to an uncertain extent1. A strongly negative aerosol forcing would imply a substantial risk because it implies a large sensitivity of the Earth’s surface temperature to anthropogenic radiative forcing2. A strongly negative aerosol forcing would also mean an acceleration of global warming when aerosol emissions are reduced to improve air quality3 and smaller than expected net carbon emissions compatible with specified temperature change targets, such as +1.5 °C4. A strongly negative aerosol forcing requires large contributions from poorly-quantified aerosol impact on clouds, because the comparatively well quantified radiative forcing of aerosol- radiation interactions has been estimated to offset only about 10% of the positive radiative forcing currently exerted by greenhouse gases1. There is comprehensive observational evidence for cloud brightening through the Twomey effect5, where anthropogenic aerosols lead to the formation of more numerous cloud droplets6-8. But general circulation models (GCMs) and studies based on satellite retrievals suggest that increases in cloud water content or cloud fraction 2 are also needed for aerosol forcing more negative than about −1.5 W/m , i.e. to offset more than half of the positive greenhouse gas forcing9,10. In this study we test the hypothesis of a substantial climate cooling effect through cloud water increases. 3 The existing evidence for the sign and magnitude of the cloud liquid water path (LWP) response to increased cloud droplet concentration is limited and conflicting11. Rain can be delayed by decreased collision-coalescence efficiency of smaller cloud droplets12,13 and this can lead to increased LWP14. But process-level modelling also suggests that LWP can decrease due to aerosol-enhanced entrainment15-17 resulting from enhanced evaporation18 and decreased sedimentation16 of smaller cloud droplets. Co-variations between LWP and aerosols in satellite data indicate both LWP increases and decreases in response to aerosol perturbations19,20. But these correlative studies are affected by cloud contamination of aerosol retrievals, aerosol swelling near clouds, wet scavenging and in-cloud aerosol processing21-23. Analyses of the relationship between cloud droplet number concentrations (CDNC) and LWP help avoid the dependence on uncertain aerosol retrievals near clouds, but rely on uncertain satellite retrievals of CDNC24. Rosenfeld et al.25 find a strongly positive relationship between CDNC and LWP. Gryspeerdt et al.26 find a non-linear relationship between CDNC and LWP, which is negative in most regions. However, such relationships do not confirm causality between LWP and CDNC changes26. Here we overcome the limitations of correlative studies by directly comparing the properties of polluted clouds perturbed by identified aerosol sources (Fig. 1) to the properties of nearby unpolluted clouds. This method ensures that the LWP response is directly linked to the CDNC perturbation and avoids the influence of meteorological covariation between aerosols and LWP. Similar analyses of temporal and spatial anomalies in LWP caused by shipping and volcanic emissions have been previously performed for ocean regions27-29. Malavelle et al.28 found that the large volcanic eruption of Holuhraun did not result in a discernible change in LWP, but they 4 could not explain why the LWP did not change. Small-scale volcano and ship tracks showed that LWP increases and decreases compensate in ocean-based low clouds to only produce a weak overall change in LWP27. But the LWP response to industrial and urban aerosols over continents, where most anthropogenic aerosol sources are located, remained unknown. We address this knowledge gap by analysing LWP responses in polluted clouds downwind of small and large cities, industries and fires (see locations in Fig. 2). Moreover, we compare LWP responses in a rich variety of clouds over land and ocean (Methods M1) and study implications of LWP changes for the effective aerosol radiative forcing. Anthropogenic pollution tracks Using Moderate Resolution Imaging Spectroradiometer (MODIS) satellite retrievals30, the properties of polluted low-level and mid-level liquid-phase clouds downwind of localized anthropogenic aerosol sources like smelters, oil refineries, coal-fired power stations, small industry towns and fires (Fig. 1, Methods M3) are compared to the properties of nearby unpolluted clouds (Methods M2, Extended Data Fig. 1). These quasi-linear polluted cloud features (Fig. 1) are referred to as pollution tracks. In total, about 2400 tracks have been identified in this study over 15 years of MODIS retrievals. Anthropogenic land-based aerosol perturbations of stratiform clouds are compared to the cloud responses in ship tracks in stratocumulus clouds, and volcano tracks (Extended Data Fig. 2) in trade-wind cumulus and stratocumulus clouds27,29. Fig. 3a shows that land-based observations sample a much wider range of clouds in terms of cloud top height than ocean-based ship and volcano tracks. The occurrence of ship tracks is largely limited to clouds no higher than 1.5 km 5 under very stable conditions. Volcano tracks occur only under a moderately wider range of conditions, in clouds up to 2.5 km high27. In contrast, industry and fire tracks occur in clouds up to 4.5 km high. In addition, the average width of ship tracks is about ten kilometres31, so ship track-like observations may not be representative of larger scale anthropogenic cloud perturbations32. Here, tracks from large scale anthropogenic cloud perturbations caused by emissions from the megacity of Moscow and a large oil production area in Kazakhstan have been found. These larger scale cloud perturbations cover thousands of square kilometres (Fig. 1). When various types of pollution tracks are combined, the sampled meteorological conditions (Extended Data Fig. 3 and 4) cover a wide range of conditions where liquid clouds occur33 (Methods M5). Occurrence of pollution tracks in clouds for various aerosol sources in various cloud regimes provides unequivocal evidence for the existence of the Twomey effect across the globe (Fig. 2). But sampling a large variety of pollution tracks also provides the opportunity to assess the global-scale response of liquid cloud water to aerosol perturbations. LWP responses to aerosols Both LWP increases and decreases are observed in all types of pollution tracks (Fig. 3b), resulting in relatively weak average LWP changes (Extended Data Table 1). This means that LWP responses to aerosol perturbations are more complex than the unidirectional increases assumed from the suppression of rain14 and observed in a limited number of pollution tracks34.
Details
-
File Typepdf
-
Upload Time-
-
Content LanguagesEnglish
-
Upload UserAnonymous/Not logged-in
-
File Pages48 Page
-
File Size-