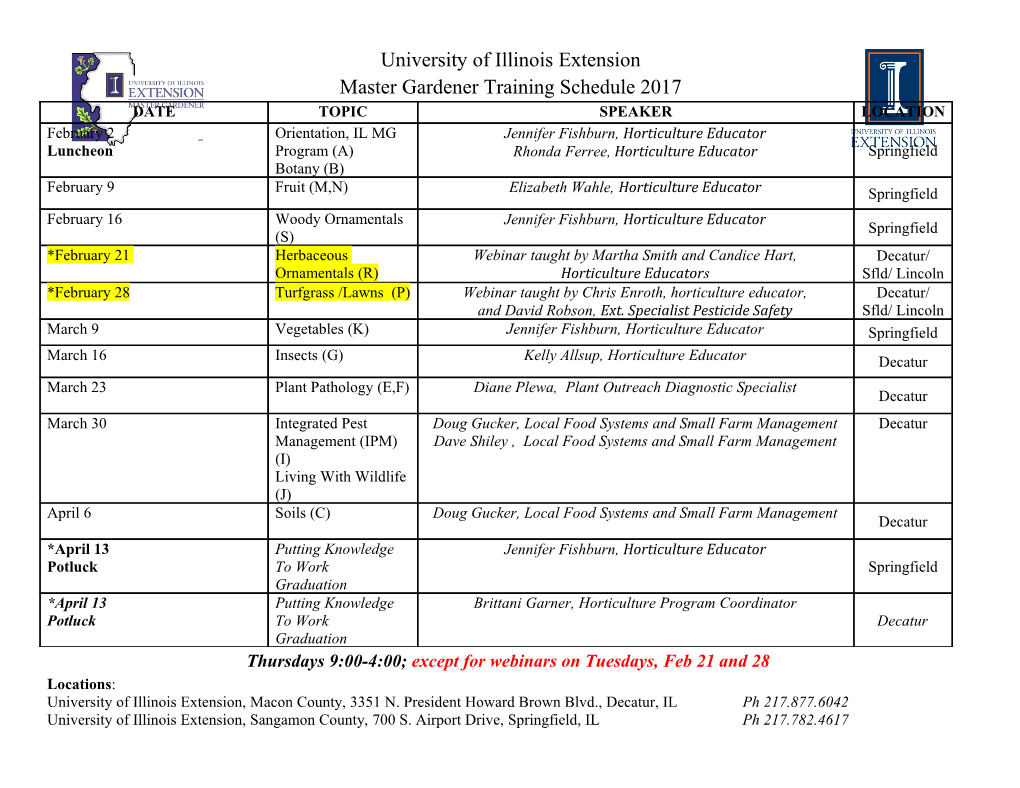
A Thesis Submitted for the Degree of PhD at the University of Warwick Permanent WRAP URL: http://wrap.warwick.ac.uk/110506 Copyright and reuse: This thesis is made available online and is protected by original copyright. Please scroll down to view the document itself. Please refer to the repository record for this item for information to help you to cite it. Our policy information is available from the repository home page. For more information, please contact the WRAP Team at: [email protected] warwick.ac.uk/lib-publications Enzymatic Synthesis of Glycosides and Oligosaccharides D.A. MacManus Submitted for the Degree of Doctor of Philosophy Department of Chemistry University of Warwick September 1991. i Acknowledgements I would like to thank Professor D.H.G. Crout for his consistent guidance, encouragement and tolerance throughout the course of this work. I would also like to thank Dr. J. Bosley and Dr. P. Critchley for their input to this work and all those at Colworth House for making the time I spent with them so enjoyable. Sincere thanks are due to my family for their kind support throughout my education. Finally, I would like to thank Stephen Shorthouse for his patience in typing this manuscript. The financial support of the SERC and Unilever Research is gratefully acknowledged. ii Declaration The work described in this thesis is the original work of the author except where acknowledgement is made to work and ideas previously described. It was carried out in the Department of Chemistry, University of Warwick, and Colworth House, Unilever Research, between October 1987 and September 1990 and has not been submitted previously for a degree at any institution. Hi Memorandum Part of this work has been published: 1. D.H.G. Crout, D.A. MacManus and P. Critchley, J. Chem. Soc., Perkin Trans / . 1990, 1865. 2. D.H.G. Crout, D.A. MacManus and P. Critchley, J. Chem. Soc. Chem. C o m m u n 1991, 376. iv Summary The f)-D-galactosidase of Escherichia coli catalysed galactosyl transfer to a variety of acceptor substrates. Transfers to simple alcohols were followed by transfers to chiral alcohols, chiral diols (bearing primary and secondary hydroxyl groups) and to a me so- diol. In particular, the regio- and stereoselective aspects of the reactions were investigated. In general, transfer to primary hydroxyl groups was favoured over transfer to secondary hydroxyl groups, but little or no preference for the transfer to specific enantiomers in a racemic mixture was observed. The results for propane-1,2-diol and butane- 1,3-diol are interpreted in terms of the possible conformations which might be adopted at the active site of the enzyme. Transfer to cii-cyclohexa-3,5-diene- 1,2-diol gave rise to two diastereoisomers. During the early stages of the reaction, a diastereoisomeric excess of ca. 80% was observed; this was reduced to ca. 20% as the yields of product reached their maximum values. Assignment of the structures of the products was based on a combination of the techniques of nuclear Overhauser enhancement and molecular modelling. a-Galactosyl transfers to lactose and cellobiose using Mortierella vinacea a-D-galactosidase were also studied. In both cases, a single trisaccharide was isolated. Spectroscopic evidence indicated that a (1-6) linkages had been formed in both cases. An acrylamide/acrylic acid polymer intended for use in enzymatic oligosaccharide synthesis was developed. The polymer was high swelling so as to allow permeation by the enzyme and could be easily stored. An attempt to introduce chiral cavities specific for certain monosaccharides was made by substituting part of the acrylamide for a boronate-containing acrylamide and carrying out the polymerisation in the presence of the monosaccharide. The success of the imprinting procedure was measured by the ability of the polymer to separate the components of a racemic mixture of the monosaccharide. The application of such "molecular imprinting" as an aid to oligosaccharide synthesis is discussed. CONTENTS CHAPTER ONE Page General Introduction: The application of enzymes in oligosaccharide synthesis 1 Leloir glycosyltransferases 3 Non-Leloir glycosyltransferases 3 Glycosidases 8 D-Mannosidases, D-glucosidases D-Galactosidases and ß-D-fructofuranosidase: mechanism of action 17 Glycosidases in synthesis 24 Reversion syntheses 26 Transglycosylation syntheses 36 Conclusion 73 CHAPTER TWO Transglycosylation studies with the ß-D-galactosidase of Escherichia coli 75 Transfer to achiral acceptors 75 Transfer to chiral acceptors 81 Transfer to cij-cyclohexa-3,5-diene-l,2-diol 100 Transfer to disaccharide acceptors 113 CHAPTER THREE Molecular imprinting as an aid to oligosaccharide synthesis 120 vii CHArifcK FOUR Page Molecular imprinting using acrylamide polymers 131 AMsopropylacrylamide polymers 132 Acrylamide-acrylic acid co-polymers 134 CHAPTER FIVE Experimental for Chapter 2 147 CHAPTER SIX Experimental for Chapter 4 169 Appendix 1 Derivation and meaning of Km 176 Appendix 2: NMR of Carbohydrates 178 Appendix 3: Calculation of values of swelling 182 Appendix 4: Boron analyses for polymers of increasing AAPB 183 REFERENCES 184 viii List of Abbreviations A Absorbance AAPB Af-Acryloyl-3-aminophenylboronic acid ACPA 4,4'-Azobis(4-cyanopentanoic acid) b r Broad Cl Chemical ionisation cm centim etre d Doublet d.e. Diastereomeric excess DMF Af.W-Dimethylformamide El Electron impact Fru Fructose Fuc L-Fucose Gal D-Galactose Gal N Ac A/-Acetyl-D-galactosamine Glc D-Glucose GIcNAc Af-Acetyl-D-glucosamine Gly Glycosyl Hex Hexosyl HPLC High performance liquid chromatography IPG Isopropylideneglycerol J Coupling constant LCST Lower critical solution temperature M Parent molecular ion m M ultiplet Man Mannose ix MBA N,AT-Methylenebisacrylamide m.p. Melting point MurNAc Muramic acid M.W. Molecular weight NMR Nuclear magnetic resonance NOE Nuclear Overhauser enhancement ONFG o-Nitrophenyl galactoside ppm Parts per million q Quartet s Singlet SDS Sodium dodecyl sulphate t Triplet TEMED TV.TV.N'.N'-Tetramethylethylenediamine TLC Thin layer chromatography U Units UDP Uridine S'-diphosphate UV Ultraviolet Xyl Xylose CHAPTER ONE General Introduction: The Application of Enzymes in Oligosaccharide Synthesis. The selective synthesis of oligosaccharides has become of increasing importance over the last few years in response to an appreciation of their role in biology.1 *23 Carbohydrate chains covalently linked to proteins and lipids are present at the periphery of all cells from every organism and in all secretions and intercellular fluids from vertebrates. It is evident that such chains in glycolipids and glycoproteins play crucial rôles in the recognition events controlling cell and tissue behaviour. Examples occur in immune systems (antigen-antibody reaction), in the reception and processing of hormones and neurotransmitters, in the recognition of molecules involved in the process of cell sorting during tissue development and in the molecular changes associated with controlled growth and differentiation. By modification of the determinants, conclusions may be drawn regarding the kind of interaction of oligosaccharides with the surface proteins. This has the potential of leading to new drugs which would, for example, prevent the adhesion of bacteria to mucosal surfaces; thus the onset of pathogenesis may be prevented by the blocking of the bacterial receptors by a carbohydrate drug. Additionally, modified glycoconjugates are indicators of diseased conditions including a number of malignancies. In this connection diagnostic kits have been developed which employ antibodies against cancer-associated carbohydrate antigens. 1 To explore and exploit the biological activity of carbohydrates there arises a need to develop synthetic procedures for oligosaccharide chains which can be employed following synthetic and biosynthetic work on oligopeptides and oligonucleotides.24 Though classical methods of carbohydrate synthesis are well developed,25*32 their inherent complexity makes this an area which provides perhaps the greatest challenge to the organic chemist. In particular, syntheses providing complete control over regio- and stereospecificity are desirable but the large number of hydroxyl groups of similar reactivity and the possibility of forming either the a - or the 0 - anomer necessitate many protection and deprotection steps. Thus a synthesis of a trisaccharide may require in excess of ten chemical steps whereas the synthesis of a decasaccharide is yet to be achieved. Where a synthesis is possible it is likely to be laborious and time-consuming, reducing both the yields and the chances of a practical large-scale synthesis. With these difficulties it is likely that oligosaccharide synthesis is the area where the application of enzymatic methods may have the greatest potential. Enzymatic methods are gaining acceptance as routine tools in organic synthesis33 and in the area of carbohydrates they offer important advantages over chemical methods. Such biotransformations preclude the need for hydroxyl group protections and provide stereospecific and often highly regiospecific syntheses. Additionally, they proceed under mild conditions in aqueous and often organic media. The appropriate enzymes are becoming more readily available and 2 immobilisations increase their lifetimes and allow their reuse. Several overviews of the types and uses of biotransformations in carbohydrate chemistry have appeared recently. 15.16.17.24 There are three main groups of enzymes dealing with oligosaccharide anabolism and
Details
-
File Typepdf
-
Upload Time-
-
Content LanguagesEnglish
-
Upload UserAnonymous/Not logged-in
-
File Pages211 Page
-
File Size-