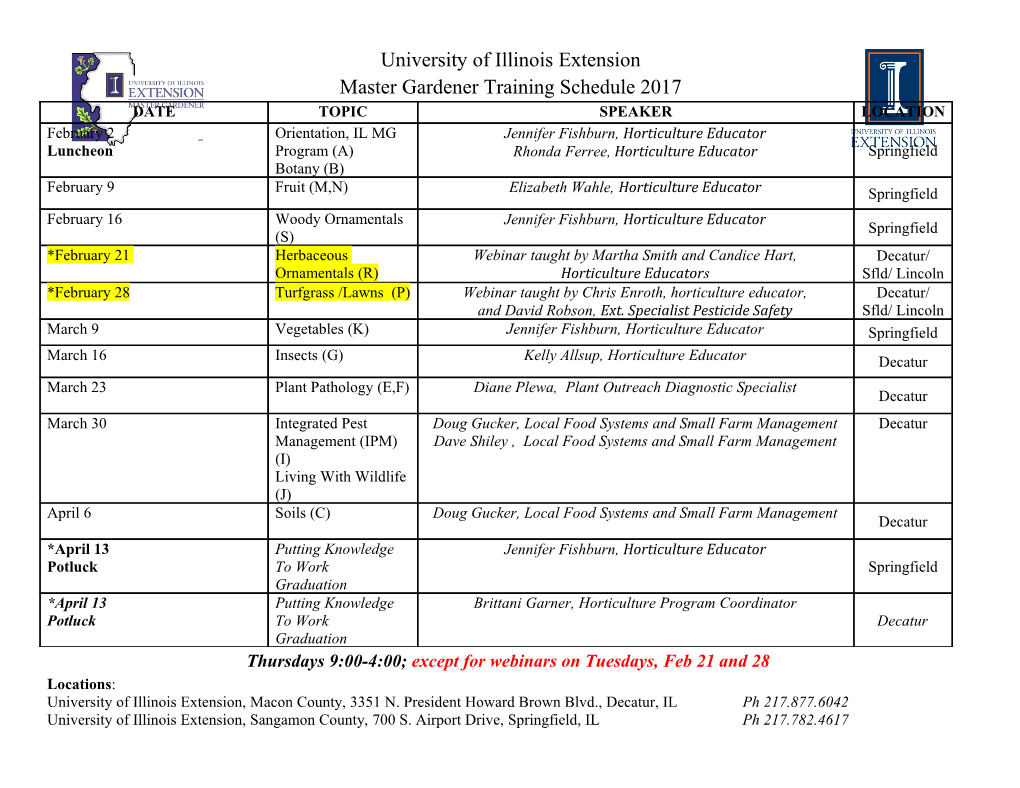
2451-3 Workshop on Interferometry and Interactions in Non-equilibrium Meso- and Nano-systems 8 - 12 April 2013 Weak quantum measurement schemes of mesoscopic currents BELZIG Wolfgang University of Konstanz Department of Physics M703 Box 703, D-78457 Konstanz Konstanz GERMANY Weak quantum measurement schemes of mesoscopic currents Wolfgang Belzig University of Konstanz Collaboration with Adam Bednorz (University of Warsaw) Kurt Franke (CERN) Christoph Bruder (University of Basel) Bertrand Reulet (University of Sherbrooke) Content • Quantum measurement, strong and weak, correlations • Markovian weak quantum measurement and quasiprobabilities, negative Wigner function, quantum violation of classical inequalities • Weak positivity of the quasiprobability for Markovian measurements; Implications for mesoscopic Bell test and time reversal symmetry. • Non-Markovian weak measurements and non-classical (squeezed) states of currents in tunnel junction • Conclusion Quantum measurement Measuring correlation function AB , ABC , ABCD Projective measurement Weak measurements allow to measure subsequently A, B, C. Which operator order? Invasive or non-invasive? Quantum description of weak (and strong) measurement (markovian schemes) Von Neumann measurement: from strong to weak Idea: couple system (Â) to pointer wavefunction (x,p) ψ = α A1 +α 2 A2 1 x igpˆAˆ ˆ gA Uint = e 2 P(x) Strong measurement (large g): Do projective measurement on well separated pointer positions, implies projection of system state gA1 or ψ f = A1 ψ f = A2 Pointer probabilities x Weak measurement (small g): projective measurement of pointer state gives almost no information, but correct average. P(x) The system state in one measurement is almost unchanged! gA2 gA1 After projection of pointer 2 ψ f ≈ α1 A1 +α 2 A2 + O(g ) Generalized measurement by POVMs Positive operator valued measure (POVM): Measurement with result A: (not necessarily orthogonal) ρˆ → ρˆ(A) = Kˆ (A)ρˆKˆ †(A) Kraus operators ˆ K(A) Probability to find A: ˆ † ˆ ∫ dA K (A)K(A) = 1 ρ(A) = Tr ρˆ(A) E.g. projection operators: ˆ ˆ -> usual projective measurement K(A) = PA Naimarks theorem: Every POVM corresponds to a projective measurement in an extended Hilbert space (including detector) Concatenation: ρˆ(A, B) = Kˆ (B)ρˆ(A)Kˆ †(B), ρ(A, B) = Tr ρˆ(A, B) ρˆ(A, B,C) = Kˆ (C)ρˆ(A, B)Kˆ †(C), ρ(A, B,C) = Tr ρˆ(A, B,C) Wiseman, Milburn, Quantum measurement; Notes on Quantum Information by J. Preskill Weak measurement using Gaussian POVM (Kraus operator) = Gaussian detector wave function (Aˆ − A)2 Phenomenological parameter λ λ −λ ˆ 4 4 λ → ∞ strong, projective measurement Kλ (A) = e 2π λ → 0 weak measurement, large error Measurement Kˆ †(A)ρˆKˆ (A) ρˆ → ρˆ = λ λ ; p(A) = Tr ⎡Kˆ †(A)ρˆKˆ (A)⎤ A p(A) ⎣ λ λ ⎦ Probability density after one measurement: Convolution of quasiprobability with classical Gaussian noise: 2 p (A) = dA′ λ / 2π e−λ(A−A′) /2q (A′) λ ∫ λ Quasiprobability after the weak measurement λ → 0 dα ˆ ˆ q (A) = e−iα ATr ⎡ei(α /2)A ρˆei(α /2)A ⎤ = Tr ⎡ρˆδ A − Aˆ ⎤ λ=0 ∫ 2π ⎣ ⎦ ⎣ ( )⎦ Corresponds here to usual probability (c.f. cyclic property of the trace) ➠ only additional Gaussian noise Bednorz, Belzig, PRL 2010 Weak measurements and quasiprobability Using a Gaussian detector wave functions (or POVM formalism) we find the probability to measure A and B as convolution of a quasiprobability with gaussian noise p(B, A) = dA'dB'g (B − B')g (A − A')q (B', A') ∫ λ λ λ probability gaussian noise quasiprobability 2 gλ (A) ~ λ / 2 exp ⎣⎡−λA / 2⎦⎤ Quasiprobability in the weak limit λ → 0 Bednorz, Belzig, PRL 2010 1 ˆ ˆ ˆ q(A, B) = dα dβe−iα A−iβBTr ⎡ρˆeiα A/2eiβBeiα A/2 ⎤ 4π 2 ∫ ⎣ ⎦ 1 AB = dAdB ABq(A, B) = Tr ⎡ρˆ Aˆ, Bˆ ⎤ ∫ 2 ⎣ { }⎦ • The quasiprobability can be equivalently defined through the quantum cumulants • In the limit of strong measurement λ → ∞ a projective measurement is obtained • In the weak limit λ → 0 we obtain the symmetrized correlation function • No backaction in the weak limit: signal ~ λ , changes to the system are ~ λ 2 Negativity of the quasiprobability can be measured by violating classical inequalities! Cumulants and correlators Quasiprobability of N weak measurements yield (after subtraction of detector noise) λ → 0 ˆ ˆ ˆ ˆ N −i(χ1A1++χN AN ) iχ A /2 iχ A /2 iχ A /2 iχ A /2 q(A ,..., A ) = d χe Tr ⎡e N N e 1 1 ρˆe 1 1 e N N ⎤ 1 N ∫ ⎣ ⎦ 1 Correlators A A A = Tr ⎡ρˆ Aˆ , Aˆ , Aˆ , Aˆ ⎤ 1→2→...→N 1 2 N N−1 ⎣⎢ { 1 { 2 { N−1 N }}}⎦⎥ 2 • weak measurement requires large detector noise • measured quantities are always “as usual” probabilities • quasiprobability after we subtract the detector noise • order of measurements matters Continuous measurement ➪ quasiprobability density functional: [Bednorz, Belzig, PRL 08] i dtχ (t )I (t ) iχ (t )Iˆ(t )dt/2e iχ (t )Iˆ(t )dt/2e q[I(t)] = Dχe ∫ Tr Te∫ ρˆTe∫ ∫ C.f. counting statistics via Keldysh orderded cumulant generating function [used e.g. by Nazarov, Kindermann (2003), Golubev, Zaikin (2005)] Negative quasiprobability to violate classical inequalities Bell inequality: using 2 detector settings for Alice (A,A’) and [Bell 1964, CHSH 1969] Bob (B,B’), all classical correlations fulfill the inequality 〈AB〉 + 〈A′B〉 + 〈AB′〉 − 〈A′B′〉 ≤ 2 2 2 2 2 with the additional assumption A = B = A′ = B′ = 1 Leggett-Garg inequality (“Bell inequality in time”) tests the question if a variable has a definite value if it is not observed (the macrorealism assumption) 〈Q(t2 )Q(t1)〉 + 〈Q(t3 )Q(t2 )〉 − 〈Q(t3 )Q(t1)〉 ≤ 1 [Leggett, Garg, 1988] 2 with the additional assumption Q (t) = 1 Weak values: Measurement protocol [Aharonov, Albert, Vaidman, PRL 88] 1) Measure A weakly (+noisy) Defines weak value of A (and an upper limit): 2) Measure B projectively 3) Select only results for some B 〈Pˆ Aˆ〉 〈Aˆ〉 = B ≤ Max A with the additional assumption B w 〈Pˆ 〉 that Max(<A>) is known B Weak positivity of the Markovian quasiprobability Second-order quantum correlations C = A A = Tr ⎡ρˆ Aˆ , Aˆ ⎤ / 2 ➠ positive definite matrix ij i j ⎣ { i j }⎦ ⎡ ⎤ A classical Gaussian distribution can reproduce the p ~ exp A C −1A / 2 quantum second-order correlations matrix g ⎢−∑ i ij j ⎥ ➠ all classical inequalities with second-order ⎣ ij ⎦ correlations only are fulfilled by the quasiprobability [Bednorz & Belzig, 2011] It is impossible to violate a classical inequality using only second order correlation functions without further assumptions! ➠ Weak positivity Note on dichotomic variables (c.f. Bell or Leggett-Garg inequalities): The additional assumption A = ±1 2 has to be experimentally verified by 4th-order correlator (A2 − 1) = 0 Quasiprobability and Wigner function Examples 1/2 −1/2 −1/4 −x2/2a2 ψ1(x)=2 ψ0(x)x/a ψ0(x)=a π e Quasiprobability and Wigner function Examples πhW− (x,p) 1 − 1 1 πhW0(x,p) 0.5 The Wigner function (for a pure state) 0.5 1 1 0 1 y y 0 -0.5 * ipy 1/2 -0.5 -1 W (x, p) = dyψ−(1x/2+ −)1ψ/4 (−x x−2/2a)2e ψ1(x)=2 ψ0(x)x/a -1 0 2 ψ0(xh)=∫ a π2 e 2 2 1 1 0 pa/h− 0 pa/h− -1 -1 -2 -1 - can take negative values 0 -1 0 1 2 -2 -2 -1 0 -2 1 2 x/a - gives correct expressions for correlators of x or p x/a Measurements of negativity: W1(0, 0) = 1/π! πhW− (x,p) W0(0, 0) = 1/π! 1 − − 1 1 Reconstruction fromπhW0 (x,p)density matrix, 0.5 0.5 1 0 assuming only 1 photon 1 is present 0 [e.g. Lvovski et al. (2001); Walraff group (2010)] -0.5 -0.5 -1 -1 0 2 2 1 1 0 pa/h− 0 pa/h− -1 -1 -2 -1 0 -1 0 1 2 -2 -2 -1 0 -2 Quasiprobability to measure x and p 1simultaneously 2 x/a x/a (but weakly) 1 −iα x−iβ p ˆ iα xˆ/2 iβ pˆ iα xˆ/2 ! q(x, p) = 2 dα dβe Tr ⎡ρe e eW1(0⎤, 0)= = =1W/π(x, p) W0(0∫ , 0) = 1/π! ⎣ ⎦ − 4π Generalizes Wigner function to arbitrary sequential measurements and arbitrary operators. Negativity of the Wigner function by cumulants Consequence of weak positivity [Bednorz, Belzig, PRA 2011]: To show the negativity of the Wigner function one needs at least 4th-order cumulants Prediction tested experimentally using single photons generated by an heralded cavity-enhanced non-degenerate parametric down-conversion. [Eran Kot, Niels Grønbech-Jensen, Bo M. Nielsen, Jonas S. Neergaard-Nielsen, Eugene S Polzik, and Anders S. Sørensen, Phys. Rev. Lett. 108, 233601 (2012)] N /2 n M = 1+ C x2 + p2 ∑n−1 2n ( ) Data Classical inequality: F = M 2 > 0 2 2 2 σ F = F − F Violation by almost 20 standard deviations! Maximal order of moment Cumulant based many- body entanglement test Cooperpair splitter 1) Supraleiter Generic entangler (e.g. superconducting junction or tunnel contact): 3) quantumQuanten− dotspunkte Detection of entanglement using spin- barriers Barrieren direkt filtered current-correlation measurements of Alice and Bob? Andreev Energieluecke N1 N2 N1 Supraleiter N2 Bob Alice [Experimental setups: Beckmann et al. a(a ') b(b ') (2004), Russo et al. (2006), Hofstetter et 2a) 2b) al. (2009,2011), Herrmann et al. (2009), Das et al. (2012)...] Supraleiter N1 Supraleiter N1 positive negative Korrelation Korrelation N2 N2 Observable: A = σ a , B = σ b ,... Bell test 1 2 Correlation C(a,b) = AB ,... Bell inequality as entanglement criterion [Bell 1964, CHSH 1969] Classical correlations fulfill C(a,b) + C(a ',b) + C(a,b ') − C(a ',b ') ≤ 2 Conditions for derivation of Bell inequality: 1.
Details
-
File Typepdf
-
Upload Time-
-
Content LanguagesEnglish
-
Upload UserAnonymous/Not logged-in
-
File Pages32 Page
-
File Size-