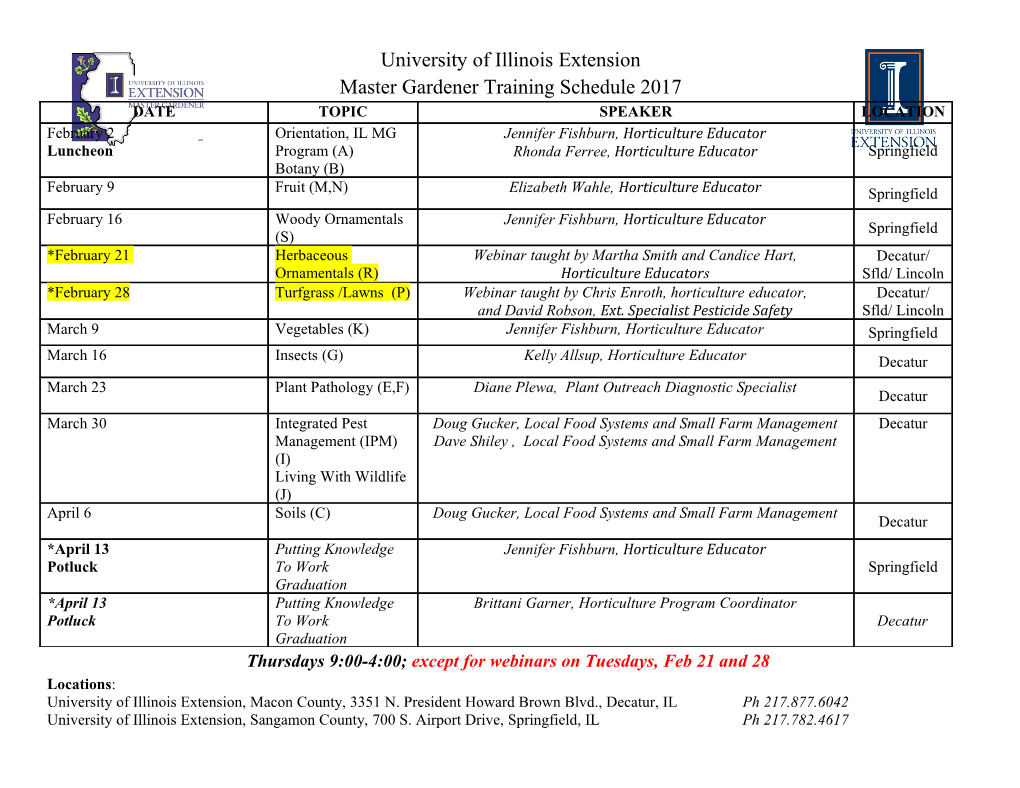
Mechanism of the hydrophobic effect in the biomolecular recognition of arylsulfonamides by carbonic anhydrase Phillip W. Snydera, Jasmin Mecinovića, Demetri T. Moustakasa, Samuel W. Thomas IIIa, Michael Hardera, Eric T. Macka, Matthew R. Locketta, Annie Hérouxb, Woody Shermanc, and George M. Whitesidesa,d,1 aDepartment of Chemistry and Chemical Biology, Harvard University, 12 Oxford Street, Cambridge, MA 02138; bNational Synchrotron Light Source, Brookhaven National Laboratory, 725 Brookhaven Avenue, Upton, NY 11973-5000; cSchrödinger, Inc., 120 West 45th Street, New York, NY 10036-4041; and dWyss Institute of Biologically Inspired Engineering, Harvard University, 60 Oxford Street, Cambridge, MA 02138 Contributed by George M. Whitesides, August 27, 2011 (sent for review June 16, 2011) The hydrophobic effect—a rationalization of the insolubility of hydrophobicity, proposed by Frank and advanced by Kauzmann, nonpolar molecules in water—is centrally important to biomolecu- Tanford, and many others, predicted that water near nonpolar lar recognition. Despite extensive research devoted to the hydro- solutes was more “ordered” than bulk water (1–5, 8–10). This so- phobic effect, its molecular mechanisms remain controversial, and called “iceberg” model rationalized thermodynamic parameters there are still no reliably predictive models for its role in protein– for partitioning at room temperature, and were compatible with ligand binding. Here we describe a particularly well-defined system both calorimetric studies of the solvation of small molecules and of protein and ligands—carbonic anhydrase and a series of struc- with structural studies of the methane clathrate-hydrates (11, 12). turally homologous heterocyclic aromatic sulfonamides—that we Although modeling studies have supported the classical mechan- use to characterize hydrophobic interactions thermodynamically ism (13, 14), neutron scattering experiments designed specifically and structurally. In binding to this structurally rigid protein, a set to probe the structure of water have failed to observe such order of ligands (also defined to be structurally rigid) shows the expected near nonpolar solutes (15, 16). gain in binding free energy as hydrophobic surface area is added. Alternative theoretical approaches for modeling the hydro- Isothermal titration calorimetry demonstrates that enthalpy deter- phobic effect—including those based on scaled-particle theory mines these increases in binding affinity, and that changes in the and its intellectual progeny (17–20)—also rationalize the unfa- heat capacity of binding are negative. X-ray crystallography and vorable entropic component of transferring nonpolar molecules molecular dynamics simulations are compatible with the proposal from a hydrophobic phase to an aqueous phase. These theories that the differences in binding between the homologous ligands propose that the accumulation of “void volume” sufficient to stem from changes in the number and organization of water mole- accommodate a nonpolar solute in water is entropically unfavor- cules localized in the active site in the bound complexes, rather than able. Although these void volume theories have been criticized CHEMISTRY (or perhaps in addition to) release of structured water from the ap- because they do not rationalize the heat capacities of partitioning posed hydrophobic surfaces. These results support the hypothesis (1), they do predict a size-dependence of the thermodynamics that structured water molecules—including both the molecules of water near nonpolar solutes: Small solutes (less than approxi- of water displaced by the ligands and those reorganized upon ligand mately 1 nm in diameter, similar in size to the nonpolar gases binding—determine the thermodynamics of binding of these studied by Frank) fit into the hydrogen bonded network of liquid ligands at the active site of the protein. Hydrophobic effects in var- water without breaking hydrogen bonds, but the solvation of lar- ious contexts have different structural and thermodynamic origins, ger solutes requires water to sacrifice hydrogen bonds to maintain although all may be manifestations of the differences in character- van der Waals contact with the solute. Water near solutes with BIOPHYSICS AND istics of bulk water and water close to hydrophobic surfaces. diameters greater than approximately 1 nm, in the void volume models, have a structure that is enthalpically less favorable than COMPUTATIONAL BIOLOGY ∣ ∣ ∣ ∣ physical-organic entropy surface water benzo-extension hydration that of bulk water (2). A body of spectroscopy studies support the prediction that molecules of water near extended hydrophobic he hydrophobic effect—the energetically favorable associa- surfaces participate in fewer hydrogen bonds than do molecules Ttion of nonpolar surfaces in an aqueous solution—often dom- of bulk water (21, 22). inates the free energy of binding of proteins and ligands (1–5). The prediction that the thermodynamics of water near solutes Frequently, increasing the nonpolar surface area of a ligand depends on the size—and implicitly on the shape—of the solute K decreases its dissociation constant ( d; i.e., increases the strength has influenced modern models of the hydrophobic effect. In of binding) (6), and simultaneously decreases its equilibrium particular, the inhomogeneous solvation theory of Lazaridis pre- constant for partitioning from a hydrophobic phase to aqueous dicts that water in chemically heterogeneous cavities (like those K solution ( P) (7). Modern, structure-guided, ligand design has that characterize the binding pockets of many proteins) possess “ ” relied upon the lock-and-key notion of conformal association structures that have free energies less favorable than the structure between the atoms of the ligand and the binding pocket of a protein; the detailed molecular basis for the hydrophobic effect, however, continues to be poorly understood (1–5). This lack of Author contributions: P.W.S. and G.M.W. designed research; P.W.S., J.M., D.T.M., S.W.T., understanding of the hydrophobic effect prevents accurate pre- M.H., E.T.M., M.R.L., A.H., and W.S. performed research; P.W.S., J.M., D.T.M., S.W.T., M.H., A.H., and W.S. analyzed data; and P.W.S., W.S., and G.M.W. wrote the paper. diction of the free energy of binding of proteins and ligands. The first, and currently most pervasive, rationale for the hydro- The authors declare no conflict of interest. phobic effect was based on studies of the thermodynamics of Data deposition: The crystallography, atomic coordinates, and structure factors have been deposited in the Protein Data Bank, www.pdb.org (PDB ID codes 3S75, 3S71, 3S78, 3S74, partitioning of nonpolar solutes from hydrophobic phases (i.e., 3S77, 3S73, 3S76, and 3S72). the gas phase or a hydrophobic liquid phase) into water. The ther- 1To whom correspondence should be addressed. E-mail: gwhitesides@gmwgroup. modynamics of partitioning of solute molecules is characterized harvard.edu. by a dominant, unfavorable entropy and an increase in heat This article contains supporting information online at www.pnas.org/lookup/suppl/ capacity of the aqueous system (8). The classical mechanism for doi:10.1073/pnas.1114107108/-/DCSupplemental. www.pnas.org/cgi/doi/10.1073/pnas.1114107108 PNAS ∣ November 1, 2011 ∣ vol. 108 ∣ no. 44 ∣ 17889–17894 of bulk water (23, 24). This theory is consistent with speculations derived from many experimental studies of molecular recognition in aqueous solution (25–31). Experimentalists have rationalized enthalpically favorable association by invoking either (i) enthal- pically favorable interactions between hosts and guests in the bound complex (so-called “nonclassical” hydrophobic effects) (4, 32, 33), or (ii) the “release” of water molecules upon associa- tion that, because of the structure of the binding pocket, adopt configurations that are enthalpically less favorable than bulk water (25, 34, 35). Many of these experimental studies, which have relied heavily on modern isothermal titration calorimeters (ITC), have also shown negative values of the heat capacity of ∘ association (ΔCp ) (26, 27, 36–40), a term that has since become the sign-post of hydrophobic interactions—even though changes in heat capacity result, in principle, from myriad structural changes that occur with association in aqueous solution (41–43). A series of recent computational studies of explicitly modeled water in the binding pockets of proteins is compatible with the rationale that water in binding pockets is less favorable in free energy than bulk water (44–50). The hydrophobic effect that determines the free energy of displacing these waters from the binding pocket appears to be quite different from the hydro- phobic effects that determine the free energy of water near small, nonpolar solutes, and that of water near large nonpolar surfaces. Although an entropy-dominated picture of hydrophobic inter- actions continues to pervade thinking in contemporary biochem- istry (51)—primarily because of Kauzmann’s plausible and un- derstandable proposal that protein folding is stabilized by the burial of hydrophobic amino acids (9)—the evidence from both theoretical and experimental studies over the last few decades paints a far more complicated picture, and one that is considerably more challenging to interpret: There is no single model that is consistent with all of the thermodynamic and structural character- izations of hydrophobic interactions, per se. Hydrophobic effects in alternative contexts may have
Details
-
File Typepdf
-
Upload Time-
-
Content LanguagesEnglish
-
Upload UserAnonymous/Not logged-in
-
File Pages6 Page
-
File Size-