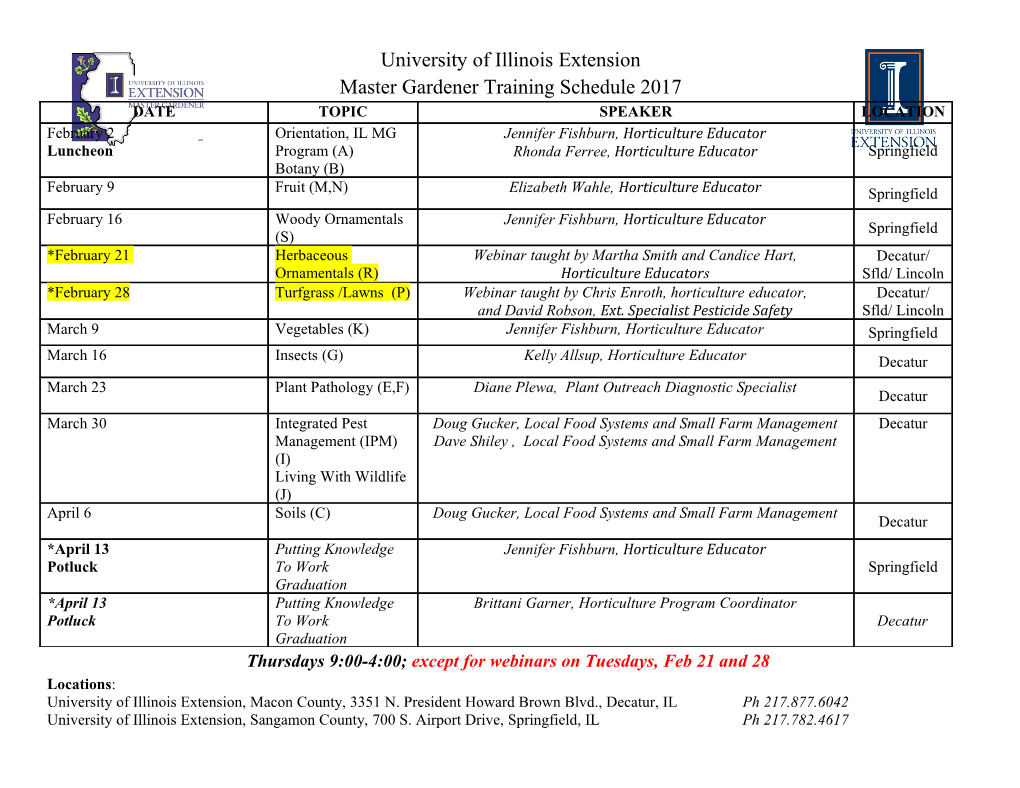
This project has received funding from the European Union’s Horizon 2020 research and innovation programme under grant agreement no 767092. Lecture 1: Integrated Circuits for Brain implants MRUT Dust Department of Engineering Integrated circuit Die Drug Aarhus University Container PZT-4 μLED 10 November 2019 Storage Cap. Target Light- Sensitive Neuron μELectrodes Associate Prof. Farshad Moradi, ICE-LAB, Aarhus University LITERATURE TO READ [1] S. A. Haddad, R. P. Houben, and W. Serdijin, “The evolution of pacemakers,” IEEE Engineering in Medicine and Biology Magazine, vol. 25, no. 3, pp. 38– 48, 2006. [2] J. D. Weiland and M. S. Humayun, “Visual prosthesis,” Proceedings of the IEEE, vol. 96, no. 7, pp. 1076–1084, 2008. [3] A. Rashidi, N. Yazdani, and A. M. Sodagar, “Fully-implantable, multichannel, microstimulator with tracking supply ribbon and energy recovery,” in 2016 38th Annual International Conference of the IEEE Engineering in Medicine and Biology Society (EMBC), Aug 2016, pp. 1818–1821. [4] J. K. Niparko, Cochlear implants: Principles & practices. Lippincott Williams & Wilkins, 2009. [5] A. L. Benabid, S. Chabardes, J. Mitrofanis, and P. Pollak, “Deep brain stimulation of the subthalamic nucleus for the treatment of parkinson’s disease,” The Lancet Neurology, vol. 8, no. 1, pp. 67–81, 2009. [6] A. M. Kuncel and W. M. Grill, “Selection of stimulus parameters for deep brain stimulation,” Clinical neurophysiology, vol. 115, no. 11, pp. 2431–2441, 2004. [7] H. Lee, K. Y. Kwon, W. Li, and M. Ghovanloo, “A power-efficient switched-capacitor stimulating system for electrical/optical deep brain stimulation,” IEEE Journal of Solid-State Circuits, vol. 50, no. 1, pp. 360–374, Jan 2015. [8] S. K. Moore, “Psychiatry’s shocking new tools [brain stimulation techniques],” IEEE spectrum, vol. 43, no. 3, pp. 24–31, 2006. [9] H. Lee, H. Park, and M. Ghovanloo, “A power-efficient wireless system with adaptive supply control for deep brain stimulation,” IEEE Journal of Solid- State Circuits, vol. 48, no. 9, pp. 2203–2216, Sep. 2013. [10] T. C. Chang, M. J. Weber, J. Charthad, S. Baltsavias, and A. Arbabian, “Scaling of ultrasound-powered receivers for sub-millimeter wireless implants,” in 2017 IEEE Biomedical Circuits and Systems Conference (BioCAS), Oct 2017, pp. 1–4 ICE-LAB, Aarhus University 3/57 LITERATURE TO READ [11] M. J. Weber, A. Bhat, T. C. Chang, J. Charthad, and A. Arbabian, “A miniaturized ultrasonically powered programmable optogenetic implant stimulator system,” in 2016 IEEE Topical Conference on Biomedical Wireless Technologies, Networks, and Sensing Systems (BioWireleSS), Jan 2016, pp. 12–14. [12] J. Charthad, T. C. Chang, Z. Liu, A. Sawaby, M. J. Weber, S. Baker, F. Gore, S. A. Felt, and A. Arbabian, “A mm-sized wireless implantable device for electrical stimulation of peripheral nerves,” IEEE Transactions on Biomedical Circuits and Systems, vol. 12, no. 2, pp. 257–270, April 2018. [13] Y. Luo, J. Wang, W. Huang, J. Tsai, Y. Liao, W. Tseng, C. Yen, P. Li, and S. Liu, “Ultrasonic power/data telemetry and neural stimulator with ookpm signaling,” IEEE Transactions on Circuits and Systems II: Express Briefs, vol. 60, no. 12, pp. 827–831, Dec 2013. [14] B. C. Johnson, K. Shen, D. Piech, M. M. Ghanbari, K. Y. Li, R. Neely, J. M. Carmena, M. M. Maharbiz, and R. Muller, “Stimdust: A 6.5 mm 3, wireless ultrasonic peripheral nerve stimulator with 82% peak chip efficiency,” in 2018 IEEE Custom Integrated Circuits Conference (CICC). IEEE, 2018, pp. 1–4. [15] S. Hosseini et al., “Multi-ring ultrasonic transducer on a single piezoelectric disk for powering biomedical implants,” in 41st Ann. Int. Con. of the IEEE Eng. in Medicine & Biology Society, July 2019, pp. 3827– 3830. [16] G. Shin, A. M. Gomez, R. Al-Hasani, Y. R. Jeong, J. Kim, Z. Xie, A. Banks, S. M. Lee, S. Y. Han, C. J. Yoo et al., “Flexible near-field wireless optoelectronics as subdermal implants for broad applications in optogenetics,” Neuron, vol. 93, no. 3, pp. 509–521, 2017. [17] T.-i. Kim, J. G. McCall, Y. H. Jung, X. Huang, E. R. Siuda, Y. Li, J. Song, Y. M. Song, H. A. Pao, R.-H. Kim et al., “Injectable, cellularscale optoelectronics with applications for wireless optogenetics,” Science, vol. 340, no. 6129, pp. 211–216, 2013. [18] K.-i. Inoue, M. Takada, and M. Matsumoto, “Neuronal and behavioural modulations by pathway-selective optogenetic stimulation of the primate oculomotor system,” Nature communications, vol. 6, p. 8378, 2015. [19] G. Gagnon-Turcotte, M. N. N. Khiarak, C. Ethier, Y. De Koninck, and B. Gosselin, “A 0.13-µm cmos soc for simultaneous multichannel optogenetics and neural recording,” IEEE Journal of Solid-State Circuits, no. 99, pp. 1–14, 2018. [20] G. Gagnon-Turcotte, Y. LeChasseur, C. Bories, Y. Messaddeq, Y. De Koninck, and B. Gosselin, “A wireless headstage for combined optogenetics and multichannel electrophysiological recording,” IEEE Transactions on Biomedical Circuits and Systems, vol. 11, no. 1, pp. 1–14, Feb 2017. ICE-LAB, Aarhus University 4/57 LITERATURE TO READ [21] J. Luo, K. Nikolic, B. D. Evans, N. Dong, X. Sun, P. Andras, A. Yakovlev, and P. Degenaar, “Optogenetics in silicon: A neural processor for predicting optically active neural networks,” IEEE Transactions on Biomedical Circuits and Systems, vol. 11, no. 1, pp. 15–27, Feb 2017. [22] G. Buzsaki, E. Stark, A. Ber ´ enyi, D. Khodagholy, D. R. Kipke, E. Yoon, ´ and K. D. Wise, “Tools for probing local circuits: high-density silicon probes combined with optogenetics,” Neuron, vol. 86, no. 1, pp. 92–105, 2015. [23] R. Erfani, F. Marefat, A. M. Sodagar, and P. Mohseni, “Modeling and experimental validation of a capacitive link for wireless power transfer to biomedical implants,” IEEE Transactions on Circuits and Systems II: Express Briefs, vol. 65, no. 7, pp. 923–927, July 2018. [24] R. Erfani and A. M. Sodagar, “Amplitude-engraving modulation (aem) scheme for simultaneous power and high-rate data telemetry to biomedical implants,” in 2013 IEEE Biomedical Circuits and Systems Conference (BioCAS), Oct 2013, pp. 290–293. [25] H. Lee and M. Ghovanloo, “An integrated power-efficient active rectifier with offset-controlled high speed comparators for inductively powered applications,” IEEE Transactions on Circuits and Systems I: Regular Papers, vol. 58, no. 8, pp. 1749–1760, Aug 2011. [26] C. Huang, T. Kawajiri, and H. Ishikuro, “A near-optimum 13.56 mhz cmos active rectifier with circuit-delay real-time calibrations for highcurrent biomedical implants,” IEEE Journal of Solid-State Circuits, vol. 51, no. 8, pp. 1797–1809, Aug 2016. [27] K. Noh, J. Amanor-Boadu, M. Zhang, and E. Snchez-Sinencio, “A 13.56-mhz cmos active rectifier with a voltage mode switched-offset comparator for implantable medical devices,” IEEE Transactions on Very Large Scale Integration (VLSI) Systems, vol. 26, no. 10, pp. 2050–2060, Oct 2018. [28] H. Cha, W. Park, and M. Je, “A cmos rectifier with a cross-coupled latched comparator for wireless power transfer in biomedical applications,” IEEE Transactions on Circuits and Systems II: Express Briefs, vol. 59, no. 7, pp. 409–413, July 2012. [29] M. J. Weber, Y. Yoshihara, A. Sawaby, J. Charthad, T. C. Chang, and A. Arbabian, “A miniaturized single-transducer implantable pressure sensor with time-multiplexed ultrasonic data and power links,” IEEE Journal of Solid-State Circuits, vol. 53, no. 4, pp. 1089–1101, April 2018. [30] H. Sadeghi Gougheri and M. Kiani, “An inductive voltage-/current-mode integrated power management with seamless mode transition and energy recycling,” IEEE Journal of Solid-State Circuits, vol. 54, no. 3, pp. 874– 884, March 2019. ICE-LAB, Aarhus University 5/57 LITERATURE TO READ [31] C. Kim, J. Park, A. Akinin, S. Ha, R. Kubendran, H. Wang, P. P. Mercier, and G. Cauwenberghs, “A fully integrated 144 mhz wirelesspower-receiver-on-chip with an adaptive buck- boost regulating rectifier and low-loss h-tree signal distribution,” in 2016 IEEE Symposium on VLSI Circuits (VLSI-Circuits). IEEE, 2016, pp. 1–2. [32] C. Kim, S. Ha, J. Park, A. Akinin, P. P. Mercier, and G. Cauwenberghs, “A 144-mhz fully integrated resonant regulating rectifier with hybrid pulse modulation for mm-sized implants,” IEEE Journal of Solid-State Circuits, vol. 52, no. 11, pp. 3043–3055, 2017. [33] R. Erfani, F. Marefat, and P. Mohseni, “A 110mhz frequency-aware cmos active rectifier with dual-loop adaptive delay compensation and ¿230 mw output power for capacitively powered biomedical implants,” in 2019 IEEE Custom Integrated Circuits Conference (CICC), April 2019, pp. 1–4. [34] A. Rashidi, K. Laursen, S. Hosseini, and F. Moradi, “An ultrasonically powered optogenetic microstimulators with power-efficient active rectifier and charge reuse capability,” in 2019 IEEE International Symposium on Circuits and Systems (ISCAS). IEEE, 2019, pp. 1–5. [35] P. Allen and D. Holberg, CMOS Analog Circuit Design. New York: Oxford University Press, 2012. [36] Rail-to-Rail, Very Fast, 2.5 V to 5.5 V, Single-Supply TTL/CMOS Comparators, Analog Devices, Jun. 2010, rev. A. [37] A. Rashidi et al., “Overvoltage protection circuits for ultrasonically powered implantable microsystems,” in 41st Ann. Int. Con. of the IEEE Eng. in Medicine & Biology Society (EMBC), July 2019, pp. 4354–4358. [38] M. Ghovanloo and K. Najafi, “Fully integrated wideband high-current rectifiers for inductively powered devices,” IEEE Journal of Solid-State Circuits, vol. 39, no. 11, pp. 1976– 1984, Nov 2004. [39] A. Rashidi, N. Yazdani, and A. M. Sodagar, “Fully-integrated, highefficiency, multi-output charge pump for high-density microstimulators,” in 2018 IEEE Life Sciences Conference (LSC), Oct 2018, pp. 291–294. [40] D. L. Miller, “Safety assurance in obstetrical ultrasound,” in Seminars in Ultrasound, CT and MRI, vol. 29, no. 2. Elsevier, 2008, pp. 156–164.
Details
-
File Typepdf
-
Upload Time-
-
Content LanguagesEnglish
-
Upload UserAnonymous/Not logged-in
-
File Pages58 Page
-
File Size-