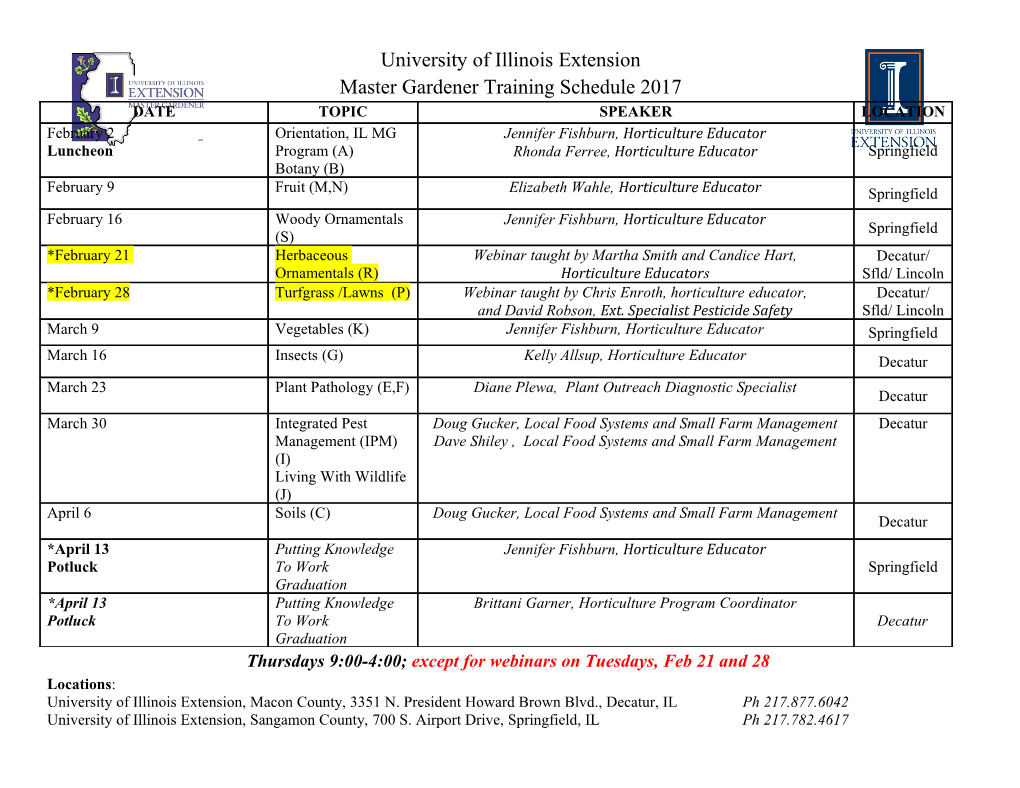
doi:10.1016/j.jmb.2011.06.009 J. Mol. Biol. (2011) 411, 321–328 Contents lists available at www.sciencedirect.com Journal of Molecular Biology journal homepage: http://ees.elsevier.com.jmb COMMUNICATION Molecular Basis of a Million-Fold Affinity Maturation Process in a Protein–Protein Interaction Daniel A. Bonsor1, Sandra Postel1, Brian G. Pierce2, Ningyan Wang3, Penny Zhu1, Rebecca A. Buonpane3, Zhiping Weng2, David M. Kranz3 and Eric J. Sundberg1⁎ 1Boston Biomedical Research Institute, 64 Grove Street, Watertown, MA 02472, USA 2Program in Bioinformatics and Integrative Biology, University of Massachusetts Medical School, Worcester, MA 01605, USA 3Department of Biochemistry, University of Illinois Urbana-Champaign, Urbana, IL 61801, USA Received 7 April 2011; Protein engineering is becoming increasingly important for pharmaceutical received in revised form applications where controlling the specificity and affinity of engineered 2 June 2011; proteins is required to create targeted protein therapeutics. Affinity increases accepted 4 June 2011 of several thousand-fold are now routine for a variety of protein engineering Available online approaches, and the structural and energetic bases of affinity maturation 12 June 2011 have been investigated in a number of such cases. Previously, a 3-million-fold affinity maturation process was achieved in a protein–protein interaction Edited by I. Wilson composed of a variant T-cell receptor fragment and a bacterial superantigen. Here, we present the molecular basis of this affinity increase. Using X-ray Keywords: crystallography, shotgun reversion/replacement scanning mutagenesis, and protein–protein interactions; computational analysis, we describe, in molecular detail, a process by protein engineering; which extrainterfacial regions of a protein complex can be rationally yeast display; manipulated to significantly improve protein engineering outcomes. X-ray crystallography; © 2011 Elsevier Ltd. All rights reserved. computational biology Modern molecular biology techniques have great- proteins now comprise one of the most important ly accelerated the use of recombinant biological and fastest-growing sectors of pharmaceutical de- molecules in the clinic.1 Accordingly, therapeutic velopment. Recombinant proteins have provided novel therapeutic options for a wide range of human diseases, including those for which no drugs *Corresponding author. Institute of Human Virology and produced by more traditional chemistry-based Department of Medicine, University of Maryland School methods exist. of Medicine, Baltimore, MD 21201, USA. E-mail address: Many proteins, however, are unsuitable as thera- [email protected]. peutic molecules in their naturally occurring state. Present addresses: D. A. Bonsor and S. Postel, Institute Protein engineering methods provide routes to of Human Virology, University of Maryland School of achieve a wide array of desired properties. One of Medicine, Baltimore, MD 21201, USA; R. A. Buonpane, the most popular and effective engineering tech- MedImmune, Gaithersburg, MD 20878, USA. niques is to subject a protein of interest to an – Abbreviations used: CDR, complementarity-determining evolutionary process.2 4 The power of protein region; TCR, T-cell receptor; SAG, superantigen; mVβ8.2, evolution derives from its ability to select variants mouse TCR Vβ8.2; SEB, staphylococcal enterotoxin B; with a desired property (in particular, improved hVβ2.1, human TCR Vβ2.1; SpeC, streptococcal pyrogenic binding affinity) from a highly diverse pool. Using exotoxin C. such methods for directed evolution, we can 0022-2836/$ - see front matter © 2011 Elsevier Ltd. All rights reserved. 322 Mechanism of a Million-Fold Affinity Maturation engineer recombinant proteins to bind a nearly that are homologous to the targeted complex in limitless repertoire of potential targets with relatively order to increase the extent and degree of affinity high specificity and affinity. maturation.17 We generated an engineered TCR Mammalian immune systems are encoded with variant named G5-8, derived from the mouse TCR natural protein engineering tools. Antibodies, as Vβ8.2 (mVβ8.2) chain, that binds to the bacterial products of the adaptive immune system, are SAG staphylococcal enterotoxin B (SEB) with a 3- exceptionally well suited for combating infectious million-fold increase in affinity relative to the wild- diseases: hypervariability and a natural affinity type mVβ8.2, with measured binding affinities of maturation process allow for the recognition of 48 pM17 and 150 μM,18 respectively. Additionally, diverse antigens;5 a constant region triggers potent we showed that G5-8 acts as an inhibitor of SEB- immune mechanisms. Antibodies have therefore mediated T-cell activation and is completely pro- been commonly used as therapeutic molecules, either tective in vivo when administered to animals in their natural state or after further engineering. challenged with a lethal dose of SEB.17 A brief Alternatives to antibodies—largely in the form overview of our semirational structure-based pro- of diverse scaffolds for the design and engineering tein engineering strategy is presented here as a of recombinant proteins that often serve as high- guide for the structural and energetic bases of the affinity steric inhibitors of deleterious protein engineered affinity maturation described below. interactions—have been developed (reviewed by Initially, following a standard directed evolution Binz et al.6 and Binz and Pluckthun7). Like strategy, we created libraries with genetic variability antibodies, these scaffold proteins are often able to in the mVβ8.2 region but without sequence length bind a wide range of target proteins. The natural changes within the targeted mVβ8.2/SEB complex protein binding partners of drug targets, although molecular interface and selected affinity-matured more restricted in their specificity than generic variants, using yeast display (Fig. 1a). Genetic scaffold proteins, provide additional alternatives for diversity was focused entirely on the CDR2 loop of protein engineering that can lead to highly effective mVβ8.2, since it forms the majority of the protein– – therapeutic proteins.8 10 protein interface with SEB and contains several hot- Protein engineering methods have been restricted spot contacts.19 From this process, we generated traditionally to amino acid sequence variations of G2-5, a variant of mVβ8.2 that binds SEB with an the initial protein architecture, and not expansion affinity of 650 pM,17 an approximate 200,000-fold or modification of the architecture itself. Recent increase relative to the wild-type complex. studies, however, have shown that engineering Subsequently extending from the G2-5 platform, strategies that dispense of natural protein architec- we followed a semirational directed evolution tures, through the recombination and rearrange- engineering strategy (Fig. 1b) that takes advantage ment of protein domains and modules, can result of the structure-based knowledge of a homologous in engineered proteins that exhibit unique molec- TCR/SAG complex—the human TCR Vβ2.1 ular recognition properties and novel functions (hVβ2.1) domain in complex with streptococcal (reviewed by Koide11). Hybrid methods that exist pyrogenic exotoxin C (SpeC). SpeC interacts with somewhere between maintaining and dispensing hVβ2.1, forming intermolecular contacts with each of the initial protein architecture, by diversifying TCRβ hypervariable loop20 (Fig. 1b, left), while SEB not only the sequence but also the length of protein contacts only the mVβ8.2 CDR2 and HV4 loops21 loops within or near the protein–protein interface, (Fig. 1a, left and middle). The CDR1 loop of hVβ2.1 have now been utilized successfully in numerous includes a noncanonical single amino acid residue molecular systems. This is yet another engineering insertion, which acts to push several residues strategy that mimics nature, especially the length C-terminal to it closer to the SpeC molecular surface diversity of complementarity-determining region to make numerous intermolecular interactions that (CDR) 3 loops in antibodies and T-cell receptors have been shown to augment the affinity of the (TCRs).12 Several groups, using as scaffold the 10th hVβ2.1/SpeC complex.22 Conversely, residues type III domain of human fibronectin, which has from the shorter CDR1 loop of mVβ8.2 are located an immunoglobin-like β-sandwich fold and CDR- at too great a distance from SEB to make specific like loops, have exploited loop length diversity to interactions (Fig. 1b, middle). achieve significant affinity gains.13–16 Here we With this more comprehensive structural under- present the detailed molecular basis of an amino standing of TCR/SAG interactions and seeking to acid residue and a loop length diversity protein functionalize the CDR1 loop of mVβ8.2 as a engineering process that, when combined, resulted meaningful contributor to increased SEB binding in a greater-than-million-fold affinity increase in an affinity, we extended the standard directed evolu- engineered TCR/superantigen (SAG) complex. tion approach (Fig. 1a, right) by generating addi- We previously used a semirational protein tional mVβ8.2 libraries that included randomized engineering strategy that incorporated structure- CDR1 loops with either one or two additional amino based knowledge concerning protein complexes acid residues relative to the wild-type sequence and Mechanism of a Million-Fold Affinity Maturation 323 (a) (b) Fig. 1. Directed evolution strategies.
Details
-
File Typepdf
-
Upload Time-
-
Content LanguagesEnglish
-
Upload UserAnonymous/Not logged-in
-
File Pages8 Page
-
File Size-