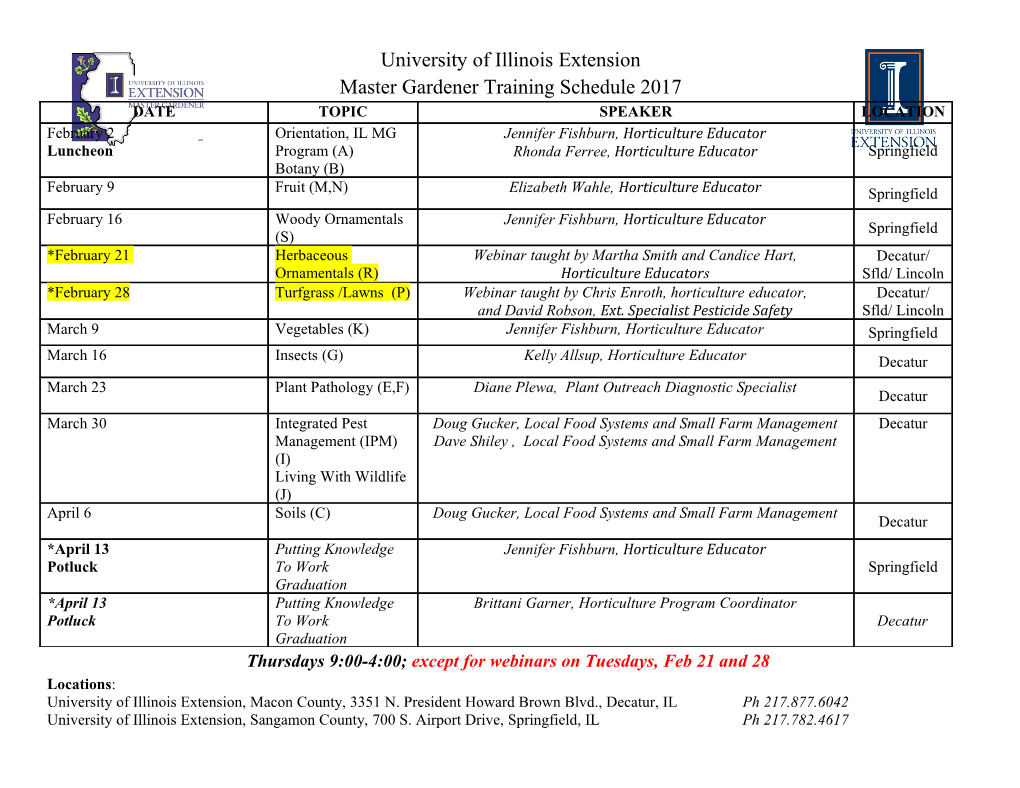
Anisotropic diffusion creep in postperovskite provides a new model for deformation at the core−mantle boundary David P. Dobsona,b,1, Alexander Lindsay-Scotta, Simon A. Hunta, Edward Baileya,2, Ian G. Wooda, John P. Brodholta,c, Lidunka Vocadloa, and John Wheelerd aDepartment of Earth Sciences, University College London, WC1E 6BT London, United Kingdom; bBayerisches Geoinstitut, Universität Bayreuth, D-95440 Bayreuth, Germany; cCentre for Earth Evolution and Dynamics, University of Oslo, 0316 Oslo, Norway; and dDepartment of Earth, Ocean and Ecological Sciences, University of Liverpool, Liverpool L69 3GP, United Kingdom Edited by Bruce Watson, Rensselaer Polytechnic Institute, Troy, NY, and approved November 13, 2019 (received for review August 26, 2019) The lowermost portion of Earth’s mantle (D″) above the core− hot regions of D″ are difficult to explain by simple flow and mantle boundary shows anomalous seismic features, such as dislocation creep. One aspect that has been relatively ignored is strong seismic anisotropy, related to the properties of the main the remarkably large anisotropy in diffusion rates along different mineral MgSiO3 postperovskite. But, after over a decade of inves- crystallographic directions predicted in ab initio calculations, tigations, the seismic observations still cannot be explained simply with up to 8 orders of magnitude difference between the fast and by flow models which assume dislocation creep in postperovskite. slow diffusion directions in postperovskite (16). This would be We have investigated the chemical diffusivity of perovskite and the largest diffusion anisotropy seen in any material, to the best postperovskite phases by experiment and ab initio simulation, and of our knowledge, and, if correct, might have significant impli- derive equations for the observed anisotropic diffusion creep. cations for the dynamics of the core−mantle boundary region. There is excellent agreement between experiments and simula- However, there have not, as yet, been any studies to test whether tions for both phases in all of the chemical systems studied. the predicted diffusion anisotropy is real. Furthermore, only one Single-crystal diffusivity in postperovskite displays at least 3 or- study has investigated the effect of the anisotropic diffusion on ders of magnitude of anisotropy by experiment and simulation the rheology of D″ (17) and only for deformation by either pure (Da = 1,000 Db; Db ≈ Dc) in zinc fluoride, and an even more extreme D = D D = D dislocation glide or climb-assisted glide. And crucially, there have anisotropy is predicted ( a 10,000 c; c 10,000 b) in the been no studies, to date, of the effect of extreme anisotropic dif- natural MgSiO3 system. Anisotropic chemical diffusivity results in fusion on lattice diffusion creep. This is because the observation EARTH, ATMOSPHERIC, AND PLANETARY SCIENCES anisotropic diffusion creep, texture generation, and a strain- of seismic anisotropy is generally thought to require dislocation weakening rheology. The results for MgSiO postperovskite 3 creep. However, the high temperatures and low stresses of the strongly imply that regions within the D″ region of Earth domi- core−mantle boundary, combined with the very small grain size nated by postperovskite will 1) be substantially weaker than re- expected in postperovskite (11, 18), would argue for diffusion gions dominated by perovskite and 2) develop a strain-induced ″ crystallographic-preferred orientation with strain-weakening rhe- creep to be the active deformation mechanism in D .Herewe ology. This leads to strain localization and the possibility to bring present a combined ab initio simulation and multianvil exper- regions with significantly varying textures into close proximity by imental study of chemical diffusion in both the perovskite and strain on narrow shear zones. Anisotropic diffusion creep there- postperovskite structures in a fluoride analog system. Fluorides fore provides an attractive alternative explanation for the com- have been shown to be a good analog for the silicate system with plexity in observed seismic anisotropy and the rapid lateral changes similar crystal chemistry in both perovskite and postperovskite in seismic velocities in D″. Significance postperovskite | D” | diffusion creep | anisotropy Earth’s core−mantle region is an important, but poorly un- he postperovskite phase (CaIrO3-type) of (Mg,Fe)(Si,Al)O3 derstood, region which drives mantle convection (and hence Tis stable at pressures above ∼120 GPa (1–3), corresponding surface processes) by heat transfer from the core to the mantle. to the D″ region of Earth’s lowermost mantle. Its strongly Seismic observations show the complexity of the region, but anisotropic elastic and rheological properties have been in- current geodynamic modeling of lowermost mantle flow is voked to explain observations of strong seismic anisotropy in hindered by an incomplete understanding of the rheology of D″ which are interpreted as requiring creep by a dislocation the main mineral, postperovskite. Here we show that post- migration process. If we can determine the active flow perovskite is strongly anisotropic in diffusion creep and hence mechanisms of postperovskite, this then offers the possibility of will show nonlinear behaviors during deformation, including interpreting the D″ seismic anisotropy in terms of convective strain weakening and localization. This will help to explain − patterns deep in Earth, and using them to map flow farther back much of the observed seismic complexity of the core mantle into deep time than current plate reconstructions allow (4–7). boundary region. This has important implications for understanding many first- Author contributions: D.P.D. designed research; D.P.D., A.L.-S., S.A.H., and E.B. performed order questions about the deep Earth, such as the degree of research; D.P.D., A.L.-S., I.G.W., L.V., and J.W. analyzed data; and D.P.D., I.G.W., J.P.B., and mixing, the ultimate fate of slabs, and the longevity of seismic J.W. wrote the paper. anomalies such as the large low-shear-velocity provinces The authors declare no competing interest. (LLSVPs). This article is a PNAS Direct Submission. In order to interpret the seismic observations, one must fully Published under the PNAS license. understand the relationships between strain and texture gener- 1To whom correspondence may be addressed. Email: [email protected]. ation in mantle rocks. Although there have been many studies 2Present address: Department of Chemical Engineering, Imperial College London, London attempting to do this for postperovskite (5, 8–15), no unique SW7 2AZ, United Kingdom. solution has been found which fits the globally observed seismic This article contains supporting information online at https://www.pnas.org/lookup/suppl/ anisotropy using a single slip system in postperovskite (e.g., ref. doi:10.1073/pnas.1914826116/-/DCSupplemental. 14). In particular, strong changes in anisotropy observed around www.pnas.org/cgi/doi/10.1073/pnas.1914826116 PNAS Latest Articles | 1of5 Downloaded by guest on September 24, 2021 structures (ref. 19 and references therein) and transforming via the same martensitic-like mechanism between the 2 phases (11). We find that, similar to the silicate system, the fluoride system also displays extreme anisotropy in chemical diffusivity in the postperovskite phase. By developing an analytical solution for strain in grains with anisotropic chemical diffusivities, we show that diffusion anisotropy will create a strain- weakening rheology, with the ultimate strain rate controlled by the fastest diffusion direction. We observe that the crystal- lographically controlled shape anisotropy of crystals deforming in lattice diffusion creep leads to crystallographic-preferred ori- entation in crystals deforming by lattice diffusion creep, and we discuss the implications of anisotropic diffusion creep for the D″ region. Anisotropic Diffusion in Postperovskite We prepared diffusion couples of NaZnF3–NaXF3 (X = Mn, Co, Fe) from presintered and polished pure end-member perovskite samples and annealed them at pressures ranging from ∼2 GPa to 14 GPa and temperatures from 823 K to 1,073 K (see SI Ap- Fig. 2. Zinc vacancy diffusivity in NaZnF3 perovskite (solid diamonds) and pendix postperovskite (solid circles), as a function of pressure at 1,073 K, predicted , section S1 for details). Recovered samples were analyzed from ab initio simulations. The diffusivity in perovskite is isotropic, but by energy-dispersive electron probe microanalysis for Zn–X postperovskite shows ∼4.5 orders of magnitude difference between the concentration gradient, and a Boltzmann−Matano analysis was fastest <100> and slowest <010> directions. The Voigt−Reuss−Hill average applied to the observed diffusion profiles. The zinc diffusivities of the postperovskite axial diffusivities (cross) is 2.8 orders of magnitude higher than perovskite diffusivity at 14 GPa and 1,073 K. This is in excellent extrapolated to pure NaZnF3 composition (equivalent to zinc self-diffusivity) are presented in Fig. 1. The perovskite diffusion agreement with the bulk diffusivity experiments (open symbols) which are coefficients at 10 GPa and below are well fitted by the Arrhenius plotted after conversion to vacancy diffusivities by a constant vacancy con- centration of 3.5 ppm. relation. −ΔH+PΔV D = D e RT [1] 0 , is also seen in the ab initio simulations of NaZnF3 postperovskite which predict a difference in bulk Zn diffusivity between perovskite 2 −1 −1 with parameters
Details
-
File Typepdf
-
Upload Time-
-
Content LanguagesEnglish
-
Upload UserAnonymous/Not logged-in
-
File Pages5 Page
-
File Size-