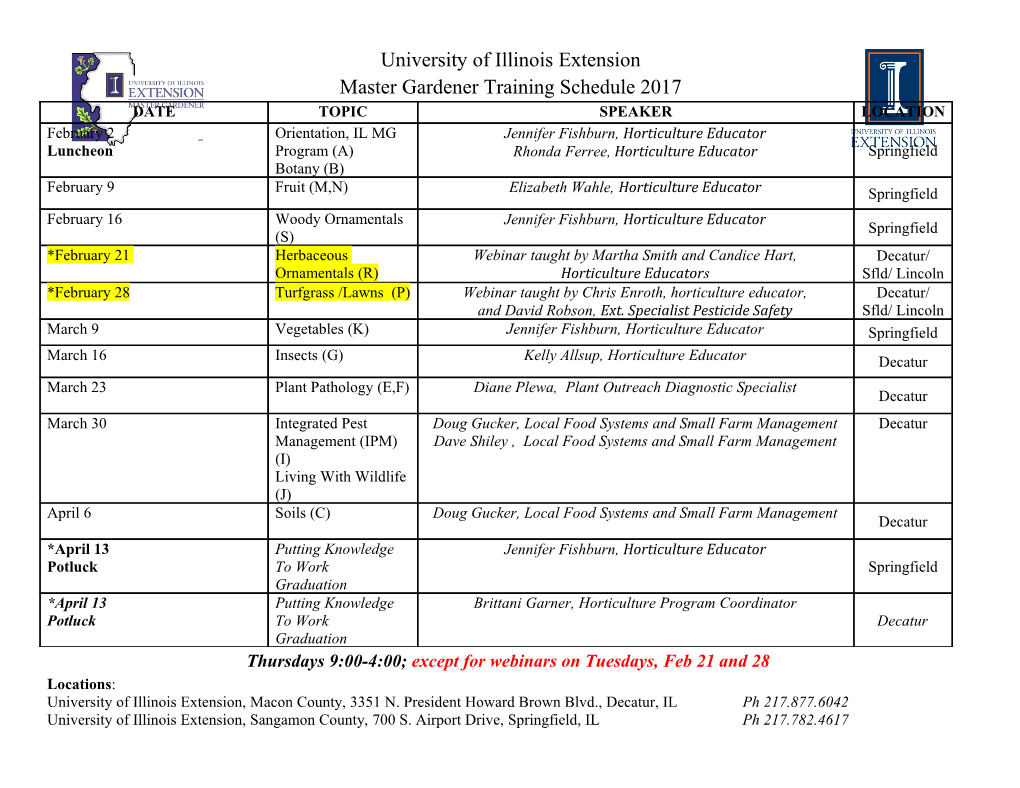
Atmos. Chem. Phys., 12, 801–813, 2012 www.atmos-chem-phys.net/12/801/2012/ Atmospheric doi:10.5194/acp-12-801-2012 Chemistry © Author(s) 2012. CC Attribution 3.0 License. and Physics Mechanisms leading to oligomers and SOA through aqueous photooxidation: insights from OH radical oxidation of acetic acid and methylglyoxal Y. Tan1,*, Y. B. Lim1, K. E. Altieri2, S. P. Seitzinger3, and B. J. Turpin1 1Department of Environmental Sciences, Rutgers University, 14 College Farm Road, New Brunswick, NJ 08901, USA 2Department of Geosciences, Princeton University, B80 Guyot Hall, Princeton, NJ 08544, USA 3International Geosphere-Biosphere Programme (IGBP), Lilla Frescativagen¨ 4a, Stockholm, Sweden *now at: Center for Atmospheric Particle Studies, Carnegie Mellon University, Pittsburgh, 15213, Pennsylvania, USA Correspondence to: B. J. Turpin ([email protected]) Received: 14 June 2011 – Published in Atmos. Chem. Phys. Discuss.: 28 June 2011 Revised: 15 November 2011 – Accepted: 9 January 2012 – Published: 18 January 2012 Abstract. Previous experiments have demonstrated that 1 Introduction the aqueous OH radical oxidation of methylglyoxal pro- duces low volatility products including pyruvate, oxalate and Large uncertainties remain in the predicted impact of sec- oligomers. These products are found predominantly in the ondary organic aerosol (SOA) on air quality, climate and hu- particle phase in the atmosphere, suggesting that methyl- man health (Kanakidou et al., 2005; Hallquist et al., 2009). glyoxal is a precursor of secondary organic aerosol (SOA). While the refined treatment of intermediate volatility organic Acetic acid plays a central role in the aqueous oxidation of compounds (IVOCs) has in some cases brought predicted or- methylglyoxal and it is a ubiquitous product of gas phase ganic aerosol mass into better alignment with measured mass photochemistry, making it a potential “aqueous” SOA pre- (Pye et al., 2010; Jathar et al., 2011), formation of SOA cursor in its own right. However, the fate of acetic acid upon through gas phase reactions followed by partitioning into aqueous-phase oxidation is not well understood. In this re- particulate organic matter (from traditional or IVOC precur- search, acetic acid (20 µM–10 mM) was oxidized by OH rad- sors) does not account for observed atmospheric SOA prop- icals, and pyruvic acid and methylglyoxal experimental sam- erties. Specifically, the oxygen-to-carbon (O/C) ratio of am- ples were analyzed using new analytical methods, in order to bient low-volatility oxygenated organic aerosol (LV-OOA, better understand the formation of SOA from acetic acid and O/C range 0.83–1.02) is higher than that formed in traditional methylglyoxal. Glyoxylic, glycolic, and oxalic acids formed smog chamber experiments (O/C range 0.3–0.4) (Aiken et from acetic acid and OH radicals. In contrast to the aque- al., 2008). Liquid water (in clouds and in aerosols) is ubiqui- ous OH radical oxidation of methylglyoxal, the aqueous OH tous in the atmosphere. In fact, globally the mass of aerosol radical oxidation of acetic acid did not produce succinic acid water exceeds the sum of all other particle species includ- and oligomers. This suggests that the methylgloxal-derived ing organic matter by 2–3 times (Liao and Seinfeld, 2005). oligomers do not form through the acid catalyzed esterifica- Thus, products of gas-phase photochemistry have greater ac- tion pathway proposed previously. Using results from these cess to liquid water than to particulate organic matter for par- experiments, radical mechanisms responsible for oligomer titioning. Laboratory and modeling experiments demonstrate formation from methylglyoxal oxidation in clouds and wet that aqueous oxidation of small water-soluble organic com- aerosols are proposed. The importance of acetic acid/acetate pounds forms SOA with high O/C ratios (Lim et al., 2005, as an SOA precursor is also discussed. We hypothesize that 2010; Carlton et al., 2006, 2008; Ervens et al., 2008; Fu this and similar chemistry is central to the daytime formation et al., 2008; El Haddad et al., 2009; Yao Liu et al., 2009; of oligomers in wet aerosols. Perri et al., 2009; Tan et al., 2009; Ervens and Volkamer, 2010; Sun et al., 2010; Zhang et al., 2010; Myriokefalitakis Published by Copernicus Publications on behalf of the European Geosciences Union. 802 Y. Tan et al.: Mechanisms leading to oligomers and SOA through aqueous photooxidation Scheme 1. Oxidation of acetic acid by OH radical (Leitner and Dore, 1997). et al., 2011). Field measurements show that aerosols are en- clude glyoxylic and glycolic acids, formaldehyde and CO2, riched in “aqueous” SOA tracers above clouds, compared to mostly through reactions 1 and 4 in Scheme 1 (Schuchmann below clouds, and at high relative humidities (Sooroshian et et al., 1985; Leitner and Dore, 1997; Benson, 1965; Russell, al., 2010). While an understanding of the aqueous chem- 1957). About 85 % of acetic acid is converted to glyoxylic istry leading to SOA formation is developing, aqueous reac- acid and subsequently to oxalic acid, the remainder is con- tion mechanisms leading to the formation of higher molec- verted to formaldehyde and formic acid. Note the formation ular weight compounds (HMWC) including oligomers, are of glycolic acid from acetic acid is not included in the Lim not well understood; and many potential aqueous SOA pre- et al. (2005) chemical mechanism, nor in the chemical mod- cursors are not yet recognized. eling of aqueous OH radical oxidation of methylglyoxal by Laboratory studies suggest that the aqueous OH radical Tan et al. (2010). The production of oxalic acid from glycolic oxidation of methylglyoxal in atmospheric waters is a source acid (via glyoxylic acid) is modest. Mechanisms leading to of SOA because this chemistry forms several organic acids the formation of HMWC remain ambiguous. (e.g., oxalate, pyruvate, glycolate) and HMWC (Altieri et Tan et al. (2010) observed that higher-MW products of al., 2008; Tan et al., 2010) that are found predominantly in methylglyoxal and OH radical become increasingly impor- the particle phase in the atmosphere (Limbeck et al., 2001; tant as organic concentrations increase from those typically Mochida et al., 2003), most likely as salts. The formation of observed in clouds to the higher concentrations found in simple carboxylic acids is generally well understood. Pyru- aerosol water. Acid catalyzed esterification was previously vic acid is the major first generation product in the aqueous proposed to explain the observed oligomer formation from OH radical oxidation of methylglyoxal (Stefan and Bolton, the methylglyoxal and OH radical reaction, and it was pro- 1999), and the subsequent oxidation of pyruvic acid yields posed that products of acetic acid and OH radicals initiated acetic acid (Carlton et al., 2006). Aqueous OH radicals are the esterification process (Altieri et al., 2008). Specifically, expected to react with acetic acid primarily by H abstrac- Altieri et al. (2008) suggested that succinic acid was pro- tion at the methyl group to produce CH2CO(OH) radicals, duced via the recombination of CH2CO2H radicals from and by H abstraction at the carboxylq group to a minor ex- acetic acid. Altieri et al. (2008) furtherq proposed that suc- tent to form the CH3CO2 radicals (Scheme 1) (Schuchmann cinic acid was oxidized by OH radicals to give hydroxy acids et al., 1985). The CH3COq 2 radicals decompose to form (e.g., lactic or hydracrylic acid), and that repeated addition CH3 radicals and are not expectedq to participate in bimolec- of monomers from hydroxy acids (e.g., C3H4O2 from hy- ularq reactions. Dissolved oxygen adds to CH2CO(OH) rad- dracrylic acid) to parent carboxylic acids (e.g., pyruvic, ox- icals, producing peroxyl radicals ( O2CHq2CO(OH)). Prod- alic, and glyoxylic acids) via esterification formed series of ucts from the bimolecular reactionq of O2CH2CO(OH) in- oligomers. Elemental composition and ion fragmentation q Atmos. Chem. Phys., 12, 801–813, 2012 www.atmos-chem-phys.net/12/801/2012/ Y. Tan et al.: Mechanisms leading to oligomers and SOA through aqueous photooxidation 803 Table 1. Comparison of acetic acid/acetate with previously identified aqueous SOA precursors. Precursor Atmospheric aqueous Aqueous phase reaction rate phase concentration constant with OH radical (M−1 s−1) Acetic acid/Acetate 0.4–245 µM 1.6×107/8.5×107 (Khare et al., 1999) (Lim et al., 2005) Glyoxal trace–276 µM 1.1×109 (Munger et al., 1995) (Lim et al., 2005) Methylglyoxal 0.02–128 µM 6.44×108 (Munger et al., 1995) (Lim et al., 2005) Glycolaldehyde 1–5 µM 1.5×109 (Bruggemann¨ et al., 2005) (Lim et al., 2005) Methyl vinyl ketone 0.02–0.2 µM 8×108 (van Pinxteren et al., 2005) (Zhang et al., 2010) Methacrolein trace–0.5 µM 1.5×109 (van Pinxteren et al., 2005) (Gligorovski and Herrmann, 2004) Phenols 0.1–30 µM 6.6×109 (Sagebiel and Seiber, 1993) (Field et al., 1982) patterns of a large number of HMWC in methylglyoxal + OH Acetic acid is a key intermediate in the aqueous oxida- radical experiments are consistent with this proposed mech- tion of methylglyoxal and a potential “aqueous” SOA pre- anism. However, these oligoesters did not form in a mixed cursor itself. Recent estimates put the global source of standard containing methylglyoxal, pyruvic acid, acetic acid, acetic acid at ∼1400 Gmol yr−1 (Paulot et al., 2011). Gas glyoxylic acid, oxalic acid and lactic acid; they only formed phase oxidation of acetyl peroxy radicals, acetone, and in the presence of OH radical. The pattern of HMWC formed propene are major sources of acetic acid. Direct emis- from the OH radical oxidation of methylglyoxal was similar sions (e.g., from biomass burning) and aqueous photochem- to that formed from the OH radical oxidation of pyruvic acid istry also contribute (Paulot et al., 2011; Warneck, 2005). (10 mM; Altieri et al., 2006). Altieri et al.
Details
-
File Typepdf
-
Upload Time-
-
Content LanguagesEnglish
-
Upload UserAnonymous/Not logged-in
-
File Pages13 Page
-
File Size-