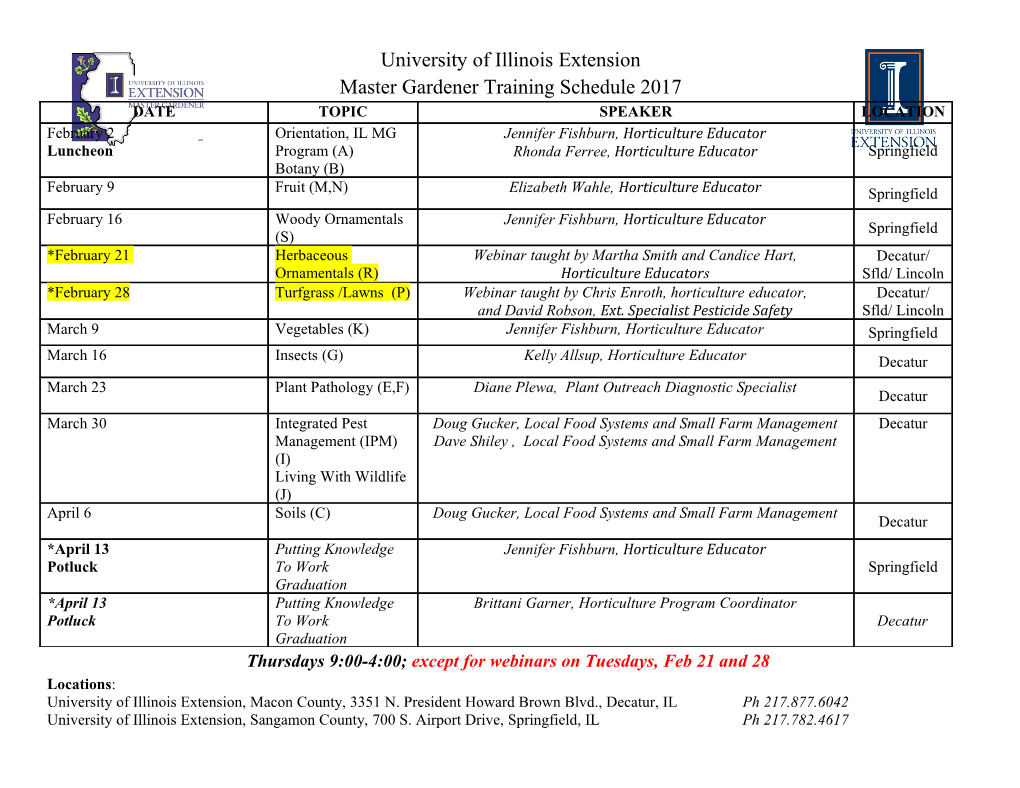
Synthetic and Mechanistic Studies into the Activation of Terminal Alkynes by a Ruthenium Acetate Complex Christine E. Welby PhD University of York Chemistry 2011 Abstract A variety of novel organometallic complexes have been easily prepared from the 2 ruthenium precursor complex 1 (cis-[Ru(κ -OAc)2(PPh3)2]. This complex itself is synthesised from readily available starting materials. The family of organometallic complexes derived from complex 1 include carbonyl, nitrosyl, carbene, vinylidene and acetylide derivatives. A number of these complexes share a common structure, as evidenced by X-Ray crystallographic studies, as well as several spectroscopic features. The differing donor/acceptor properties of these ancillary ligands may be detected by a comparison of these common structural and spectroscopic features. The addition of terminal alkynes (HC≡CR) to complex 1 results in the rapid 1 2 formation of vinylidene complexes [Ru(κ -OAc)(κ -OAc)(=C=CHR)(PPh3)2], whilst the addition of propargylic alcohols (HC≡C{R}{R‟}OH) results in the equally rapid formation of hydroxy-vinylidene complexes [Ru(κ1-OAc)(κ2- OAc)(=C=CHC{R}{R‟}OH)(PPh3)2]. The rapid formation of these vinylidene complexes has been attributed to the assistance provided by a coordinated acetate ligand. A comprehensive DFT study has shown how the acetate ligand is able to behave as both acid and base, acting as a proton shuttle, to facilitate the alkyne-to- vinylidene tautomerisation. This process has been termed a Ligand-Assisted Proton Shuttle (LAPS), which is related to the AMLA/CMD process that also facilitates the formation of acetylide complexes The novel hydroxy-vinylidene complexes are not observed to undergo the typical dehydration process to form an allenylidene derivative. Instead, a conversion to a carbonyl complex with the concomitant formation of an alkene is observed. An experimental investigation using 18O-labelling, kinetic and stoichiometric studies into the mechanism of this conversion has once more demonstrated the chemically non-innocent behaviour of an acetate ligand. i List of Contents Chapter 1: Introduction 1.1: Preamble 1 1.2: Bonding in Transition-Metal Vinylidene and Allenylidene Complexes 4 1.3: Synthesis of Transition-Metal Vinylidene and Allenylidene Complexes 13 1.3.1: Addition of a Terminal Alkyne: Vinylidene complexes 13 1.3.2: Addition of a Terminal Alkyne: Allenylidene complexes 17 1.3.3: Alternative Preparations of Vinylidene and Allenylidene 22 Complexes 1.3.3.1: Alternative Preparations of Transition-metal Vinylidene 22 Complexes 1.3.3.2: Alternative Preparations of Transition-metal Allenylidene 25 Complexes 1.4: Mechanism of Formation of Transition-Metal Vinylidene 26 and Allenylidene complexes 1.4.1: Path I – A concerted 1,2-hydrogen shift 27 1.4.2: Path II – Oxidative Addition and 1,3-hydrogen shift 29 1.4.3: Path III – Alkyne Insertion into Hydride ligand 33 1.5: Reactivity of Transition-Metal Vinylidene and Allenylidene complexes 35 1.5.1: Formation of Oxacyclocarbene complexes 35 1.5.2: Allenylidene to Indenylidene Rearrangement 39 ii 1.6: Catalytic Applications of Transition-Metal Vinylidene and 41 Allenylidene complexes 1.6.1: Ruthenium Vinylidene complexes 42 1.6.2: Ruthenium Allenylidene complexes 47 1.7: Conclusions 50 1.8: Project Aims 51 1.9: References 54 Chapter 2: Synthesis and Characterisation of novel Ruthenium Vinylidene complexes 2.1: Introduction 64 2.2: Reaction of 1 with HC≡CPh 65 2.3: Reaction of 1 with additional alkynes 74 2.4: Conclusions 82 2.5: References 83 2.6 Experimental 86 Chapter 3: Synthesis and Characterisation of novel Ruthenium Acetylide complexes 3.1: Introduction 97 iii 3.2: Unsuccessful attempts to deprotonate 2a and 2c 98 1 2 3.3 Preparation of [Ru(κ -OAc)(κ -OAc)(CO)(PPh3)2] 101 1 2 3.4 Preparation of [Ru(κ -OAc)(κ -OAc)(NO)(PPh3)2]BF4 107 3.5: Successful syntheses of acetylides 111 2 3.5.1: Synthesis of [Ru(κ -OAc)(C≡CPh)(CO)(PPh3)2] 112 2 3.5.2: Synthesis of [Ru(κ -OAc)(C≡CPh)(NO)(PPh3)2]BF4 119 3.6: Conclusions 124 3.7 References 126 3.8 Experimental 129 Chapter 4: Mechanistic Studies on the Formation of Ruthenium Vinylidene and Acetylide complexes 4.1: Introduction 140 4.2: Experimental study of formation of 2a 141 4.3: Computational (DFT) study of 2a formation 150 2 4.3.1: Model (i) Ru(κ -OAc)2(PH3)2 + HC≡CMe 153 2 4.3.2: Model (ii) Ru(κ -OAc)2(PPh3)2 + HC≡CPh 157 4.4: Experimental investigation into the mechanism of the formation 161 of 6 and 7 4.5: Computational investigation into the formation of 6 166 4.6: Conclusions 170 4.7: References 172 iv 4.8: Experimental 176 Chapter 5: Synthesis and Characterisation of novel Ruthenium Hydroxy- Vinylidene complexes 5.1: Introduction 182 5.2: Synthesis and Characterisation of 184 1 2 Ru(κ -OAc)(κ -OAc)(=C=CHC(R)(R’)OH)(PPh3)2 1 2 5.3: Reactivity of [Ru(κ -OAc)(κ -OAc)(=C=CHC(R)(R’)OH)(PPh3)2] 196 5.4 Catalytic Studies 199 5.4.1 Catalytic conversion of Propargylic Alcohols to Alkenes 200 5.4.2 Catalytic conversion of Propargylic Alcohols to 208 β-oxopropyl esters 5.5: Conclusions 212 5.6: References 213 5.7: Experimental 219 Chapter 6: Mechanistic Studies on the Decarbonylation of Propargylic Alcohols 6.1: Introduction 243 6.2: Identification of Intermediates by Stoichiometric Reactions 244 6.2.1: Formation of a phenoxy-vinylidene complex and its 244 subsequent conversion to phenyl acetate 2 and [Ru(κ -OAc)(CH=CH2)(CO)(PPh3)2] 6.2.2: Further examples of substituted vinylidene complexes 248 v and their subsequent conversion to 2 [Ru(κ -OAc)(CH=CH2)(CO)(PPh3)2] 2 6.2.3: Formation of [Ru(κ -OAc)(COCH=CH2)(CO)(PPh3)2] by 250 2 addition of CO to [Ru(κ -OAc)(CH=CH2)(CO)(PPh3)2] 2 6.2.4: Addition of acetic acid to [Ru(κ -OAc)(CH=CH2)(CO)(PPh3)2] 253 2 and [Ru(κ -OAc)(COCH=CH2)(CO)(PPh3)2] 1 6.2.5: Formation of [Ru(κ -OAc)(=C(OAc)=CHPh)(CO)(PPh3)2] 256 6.2.6: Mechanistic Implications of Complexes 10, 11 and 12 262 6.3: Stoichiometric Reactions with a Benzoate complex 265 6.4: 18O-labelling study 278 6.5: Kinetic study 284 6.6: DFT study 293 6.7: Conclusions 303 6.8: References 305 6.9: Experimental 308 Chapter 7: Synthesis and Characterisation of novel Ruthenium Oxacyclocarbene complexes. 7.1: Introduction 331 7.2: Synthesis and Characterisation of Five- Six and 333 Seven-Membered Oxacyclocarbene Complexes 7.3: Conclusions 342 vi 7.4: References 343 7.5: Experimental 345 Chapter 8: A Comparison of Structural and Spectroscopic Features of Acetate- 2 Containing Complexes derived from [Ru(κ -OAc)2(PPh3)2]. 8.1: Introduction 353 8.2: Structure 8A 357 8.3: Structure 8B 366 8.4: Thesis Conclusions 370 8.5: References 374 Abbreviations 375 List of References 379 vii List of Tables and Illustrations Chapter 1: Introduction Figure 1.1.1: The vinylidene and allenylidene ligands coordinated to a 1 transition-metal complex. Figure 1.1.2: Comparative energies of vinylidene and acetylene tautomers. 1 Figure 1.1.3: Three tautomeric forms of allenylidene. 2 Figure 1.1.4: The first reported examples of allenylidene complexes made in 3 1976. Figure 1.1.5: Formation of vinylidene and allenylidene complexes. 3 Figure 1.2.1: Simplified molecular orbital diagram for a transition-metal 5 vinylidene complex. Figure 1.2.2: Reaction of a vinylidene complex with water and an alcohol. 5 Figure 1.2.3: Summary of Werner‟s additions of electrophiles to 6 i [RhCp(=C=CHR)(L)] (L = P Pr3; R = H, Me, Ph). Figure 1.2.4: Literature examples of electrophilic addition to the metal centre 7 of a vinylidene complex in preference to Cβ. Figure 1.2.5: Vertical and Horizontal orientations of a vinylidene ligand. 8 Figure 1.2.6: Simplified molecular orbital diagram for a transition-metal 10 allenylidene complex. Figure 1.2.7: Addition of phosphine nucleophiles to 11 5 [Ru(=C=C=CPh2)(η -C9H7)L2]PF6 Figure 1.2.8: Three resonance structures that contribute to the bonding of an 12 allenylidene ligand. Figure 1.3.1.1: Formation of a vinylidene complex upon addition of HC≡CR. 13 viii Figure 1.3.1.2: Synthesis of ruthenium-vinylidene complexes by halide ligand 14 loss. Figure 1.3.1.3: Formation of vinylidene complexes by addition of terminal 15 alkynes. Figure 1.3.1.4: Competing reaction pathways in the formation of vinylidene 15 complexes from [RuClCp*(PPh3)2] Figure 1.3.1.5: Proposed mechanism for the formation of 16 t [RuCl2(=C=CH Bu)(PPh3)2] Figure 1.3.1.6: Formation of Ru- and Ir-vinylidene complexes utilising a 17 hemi-labile ligand. Figure 1.3.2.1: Selegue‟s preparation of an allenylidene complex from a 18 propargylic alcohol substrate. Figure 1.3.2.2: Isomerisation of a propargylic alcohol to either an allenylidene 18 or vinylvinylidene. Figure 1.3.2.3: Formation of an allenylidene complex assisted by acidic 19 alumina. Figure 1.3.2.4: Gimeno and Cadierno‟s work investigating the equilibrium 20 between allenylidene and vinylvinylidene complexes. Figure 1.3.2.5: Equilibrium between vinylvinylidene and allenylidene 21 complexes of [Ru(Cp)(PH3)2]. Figure 1.3.2.6: Fischer‟s alternative synthesis to allenylidene complexes of 21 Cr and W. Figure 1.3.3.1.1: Interconversion of vinylidene and acetylide ligands by 22 protonation/deprotonation.
Details
-
File Typepdf
-
Upload Time-
-
Content LanguagesEnglish
-
Upload UserAnonymous/Not logged-in
-
File Pages441 Page
-
File Size-