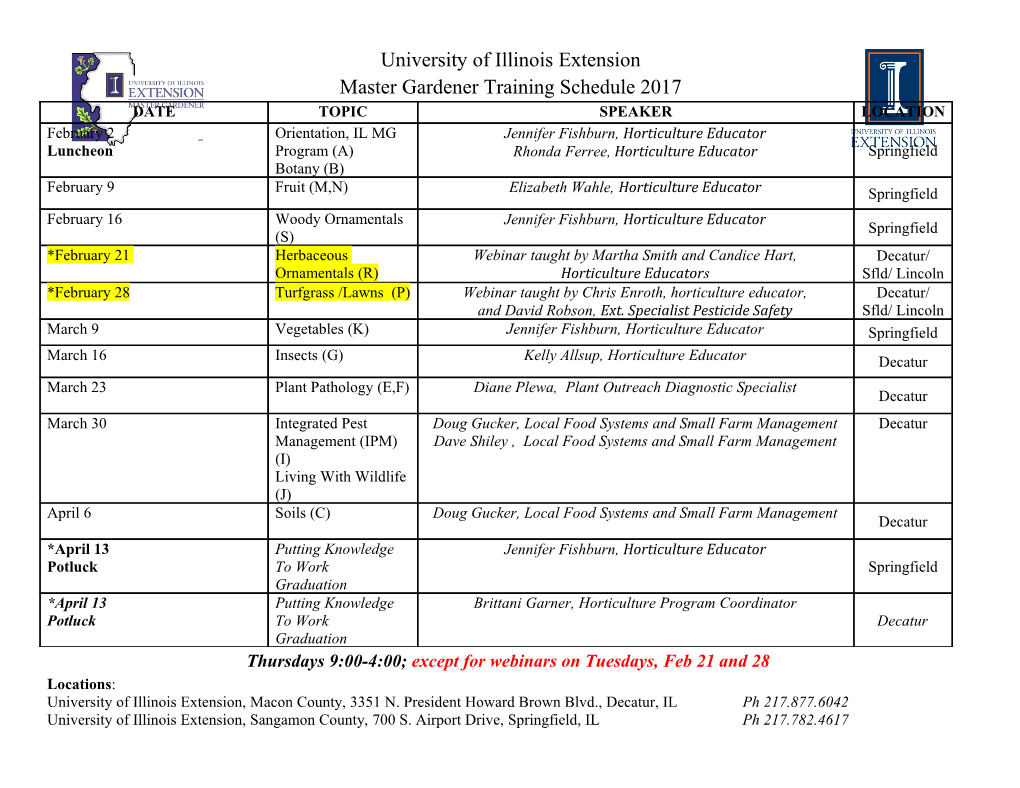
Embryo fossilization is a biological process mediated by microbial biofilms Elizabeth C. Raffa,b,1, Kaila L. Schollaerta, David E. Nelsona, Philip C. J. Donoghuec, Ceri-Wyn Thomasc, F. Rudolf Turnera, Barry D. Steina, Xiping Dongd, Stefan Bengtsone, Therese Huldtgrene,f, Marco Stampanonig,h Yin Chongyui, and Rudolf A. Raffa,b,1 aDepartment of Biology and Indiana Molecular Biology Institute, Indiana University, Bloomington, IN 47405; bSchool of Biological Sciences, University of Sydney, Sydney NSW 2006, Australia; cDepartment of Earth Sciences, University of Bristol, Bristol BS8 1RJ, United Kingdom; dDepartment of Earth and Space Sciences, Peking University, Beijing 100871, Peoples Republic of China; eDepartment of Palaeozoology, Swedish Museum of Natural History, SE-104 05 Stockholm, Sweden; fDepartment of Geology and Geochemistry, Stockholm University, SE-106 91 Stockholm, Sweden; gSwiss Light Source, Paul Scherrer Institut, 5232 Villigen, Switzerland; hInstitute for Biomedical Engineering, University and ETH Zu¨rich, Zu¨rich, Switzerland; and iInstitute of Geology, Chinese Academy of Geological Sciences, Beijing 100864, Peoples Republic of China Communicated by James W. Valentine, University of California, Berkeley, CA, October 9, 2008 (received for review August 19, 2008) Fossilized embryos with extraordinary cellular preservation appear in can occur. We show that embryo taphonomy is the product of 3 the Late Neoproterozoic and Cambrian, coincident with the appear- distinct processes. First, and most rapid, is autolysis, the self- ance of animal body fossils. It has been hypothesized that microbial destruction of cells within hours under aerobic conditions by processes are responsible for preservation and mineralization of their own lytic enzymes (11, 12). Thus, preservation sufficient for organic tissues. However, the actions of microbes in preservation of potential fossilization with preservation of tissue and cell struc- embryos have not been demonstrated experimentally. Here, we ture requires that there were conditions in ancient marine show that bacterial biofilms assemble rapidly in dead marine embryos environments that provided a rapid block to autolysis on death and form remarkable pseudomorphs in which the bacterial biofilm of the embryo. Second, during decay, bacteria invade and replaces and exquisitely models details of cellular organization and consume the embryo. We found that biofilms formed by invading structure. The experimental model was the decay of cleavage stage bacterial communities maintain embryo form by pseudomor- embryos similar in size and morphology to fossil embryos. The data phing biological structures. Third, bacteria also produce changes show that embryo preservation takes place in 3 distinct steps: (i) in embryo chemistry that affect mineralization potential; thus, blockage of autolysis by reducing or anaerobic conditions, (ii) rapid rapid formation of fine-grain mineralization may be catalyzed by formation of microbial biofilms that consume the embryo but form a biofilm bacteria (8). We identify major bacterial taxa involved replica that retains cell organization and morphology, and (iii) bac- with the 2 latter processes. terially catalyzed mineralization. Major bacterial taxa in embryo decay biofilms were identified by using 16S rDNA sequencing. Decay Results processes were similar in different taphonomic conditions, but the Autolysis Versus Bacterial-Mediated Preservation. Cell boundaries composition of bacterial populations depended on specific condi- and cellular structures are rapidly degraded after death if tions. Experimental taphonomy generates preservation states similar autolysis is not blocked (Fig. 1C). Thus, for fossilization of to those in fossil embryos. The data show how fossilization of soft embryo structures to occur, the conditions for fossilization must tissues in sediments can be mediated by bacterial replacement and block autolysis, which experimentally can be prevented by re- mineralization, providing a foundation for experimentally creating ducing conditions (produced by addition of 100 mmol of -mer- biofilms from defined microbial species to model fossilization as a captoethanol) (11, 12), or anaerobic conditions (see Materials biological process. and Methods). Evidence that compatible conditions obtained during the initial stages of metazoan fossilization is apparent in bacterial ͉ developmental evolution ͉ metazoan origins ͉ the sediments from which fossilized embryos have been recov- taphonomy ͉ Cambrian ered (13). Bacterial growth, but not autolysis, could be blocked by the broad-spectrum antibiotic rifampicin. When both autol- ossilized embryos from the Late Proterozoic and Cambrian ysis and microbial activity were blocked, embryos did not Fprovide the only direct insight into embryology during the undergo any perceivable structural degradation in either aerobic emergence of animal phyla (1–5). These fossils exhibit excep- or anaerobic conditions. Bacterial action is therefore the primary tional preservation of cell geometry and cytological structure. mediator of all taphonomic processes except autolysis. However, the mechanism of fossilization is not well understood. It has been hypothesized from fossil evidence that microbial Bacterial Biofilms Preserve Details of Cellular Structure by Generating processes are responsible for preservation and mineralization of Pseudomorphs of Embryos. Biofilms are 3-dimensional aggrega- organic tissues, and experimental studies have revealed insights tions of bacteria within a cohesive hydrated exopolysaccharide into mineralization conditions (6–9). However, how bacteria and matrix (14). In our experiments, embryo structure provides the biofilms function in the preservation of embryo structure has not template on which bacterial biofilms form. The biofilm replaces, been observed and experimentally studied. We have carried out replicates, and stabilizes the complete morphology of the con- experimental taphonomy by using early stage embryos from the sumed embryo in fine detail. The effects of experimental Australian sea urchin Heliocidaris erythrogramma (Fig. 1 A and B) that resemble Neoproterozoic and Cambrian fossil embryos in general features of cell morphology and size (10). Our results Author contributions: E.C.R., D.E.N., P.C.J.D., and R.A.R. designed research; E.C.R., K.L.S., P.C.J.D., C.-W.T., F.R.T., B.D.S., X.D., S.B., T.H., M.S., and R.A.R. performed research; P.C.J.D., illuminate the processes of decay, preservation, and mineraliza- X.D., S.B., M.S., and Y.C. contributed new reagents/analytic tools; E.C.R., K.L.S., D.E.N., tion of animal embryos, as well as biases in preservation that may P.C.J.D., C.-W.T., T.H., M.S., and R.A.R. analyzed data; and E.C.R., D.E.N., P.C.J.D., S.B., and influence interpretations of developmental mode (10–12). R.A.R. wrote the paper. Here, we report experiments in which we examined decay The authors declare no conflict of interest. under a variety of aerobic and anaerobic conditions to determine 1To whom correspondence may be addressed. E-mail: [email protected] or raffr@ the conditions under which fossilization with the fine preserva- indiana.edu. tion of cellular morphology that is evident in some fossil embryos © 2008 by The National Academy of Sciences of the USA 19360–19365 ͉ PNAS ͉ December 9, 2008 ͉ vol. 105 ͉ no. 49 www.pnas.org͞cgi͞doi͞10.1073͞pnas.0810106105 Downloaded by guest on September 30, 2021 GEOLOGY EVOLUTION Fig. 1. Taphonomic patterns in embryos. Scanning electron micrographs. (A–J) Experimental taphonomy of Heliocidaris erythrogramma embryos. All embryos except C killed as in A.(A–G) Freeze-fractured. (A) Embryo killed during second cleavage with seawater containing 100 mmol of -mercaptoethanol (BME); fixed at 18 h. Cell structure and organization are intact: fe, fertilization envelope; pv, perivitelline space; cc, dense cortical cytoplasm; ic, inner cytoplasm with numerous lipid vesicles (lipids extracted by sample preparation). (B) Cytoplasmic layers in similar control embryo. (C) Two-cell embryo killed by 10 min in seawater containing 1% NH4 (11); fixed after 24 h in seawater containing rifampicin. Fertilization envelope is intact, but autolysis has destroyed internal structure. (D) Five days in standard aerobic decay conditions (Materials and Methods). Bacteria have consumed the embryo, but resulting biofilms preserve cell organization. Fertilization envelope is closely apposed to embryo surface. (E) Three days decay in seawater with no reducing agents; bacterially replicated cytoplasmic layers retain morphology and lipid vesicles (arrows). (F) Embryo E, internal cytoplasm with extracellular matrix of the bacterial biofilm visible at lipid vesicle surface (arrows). (G) Embryo D, internal cytoplasm showing extracellular matrix fibers of the bacterial biofilm (arrows). (H) Fertilization envelope after 5 days in standard anaerobic decay conditions (Materials and Methods); anaerobic biofilm forming. (i) Dense biofilm on embryo surface after 5 days anaerobic decay with anaerobic mud inoculum (Materials and Methods). Filamentous bacterium extends 35 m (arrows). (J) Four days anaerobic decay with anaerobic mud inoculum. Fertilization envelope partly covers the embryo surface (s). Filamentous bacterium (arrow) exceeds 300 m. (K–M) Lower Cambrian phosphorite-preserved fossil embryos from Kuanchuanpu, China. (K) Embryo partly covered by presumptive fertilization envelope; exposed surface has filamentous structures (arrow). (L) Embryo K surface: 100- to 200-m filaments among mineral
Details
-
File Typepdf
-
Upload Time-
-
Content LanguagesEnglish
-
Upload UserAnonymous/Not logged-in
-
File Pages6 Page
-
File Size-