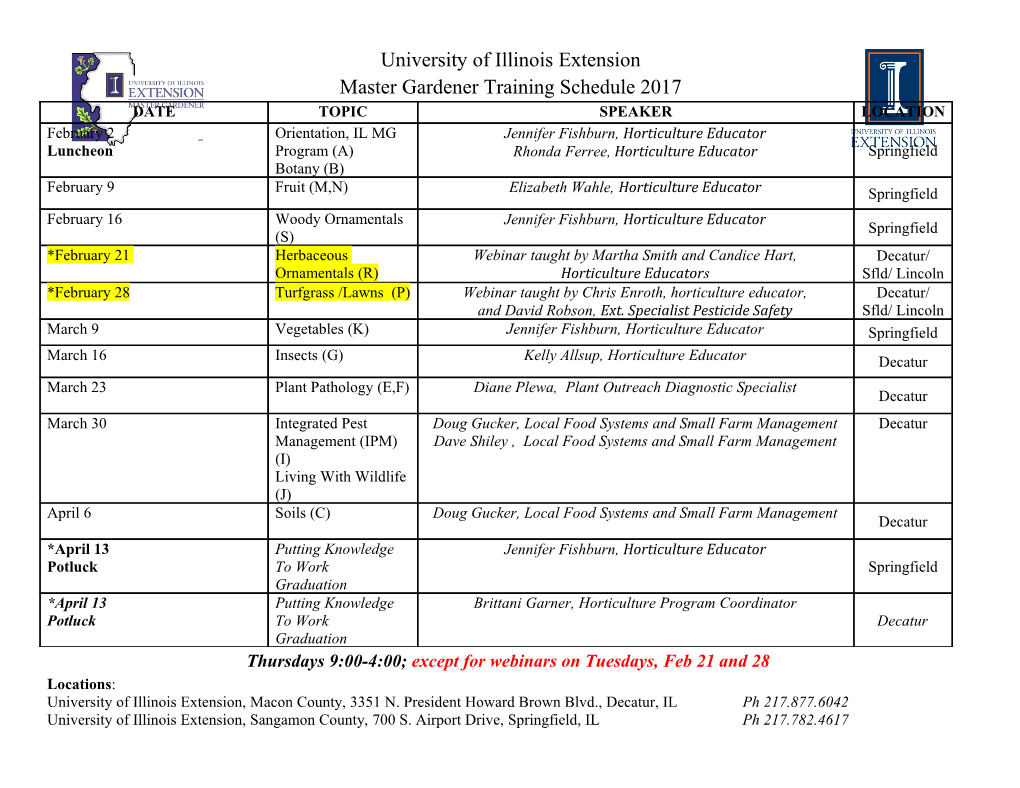
bioRxiv preprint doi: https://doi.org/10.1101/286724; this version posted March 22, 2018. The copyright holder for this preprint (which was not certified by peer review) is the author/funder. All rights reserved. No reuse allowed without permission. 1 Temperature explains broad patterns of Ross River virus transmission across Australia 2 3 Marta S. Shocket1* ([email protected]), Sadie J. Ryan2,3,4 ([email protected]), and Erin A. 4 Mordecai1 ([email protected]) 5 6 1Department of Biology, Stanford University, 371 Serra Mall, Stanford, CA, USA 7 2Department of Geography, University of Florida, Turlington Hall, Gainesville, FL, USA 8 3Emerging Pathogens Institute, University of Florida, Gainesville, FL, USA 9 4School of Life Sciences, College of Agriculture, Engineering, and Science, University of 10 KwaZulu Natal, Scottsville, KwaZulu Natal, South Africa 11 12 Statement of authorship: EAM conceived of and designed the study. MSS collected data, 13 performed statistical analyses, and wrote the first draft of the manuscript. SJR performed 14 geographic analyses. All authors revised the manuscript. 15 Data accessibility statement: Upon acceptance, all data will be archived in a public repository. 16 17 Keywords: Aedes vigilax, arbovirus, Culex annulirostris, mosquito-borne disease, infectious 18 disease, Murray Valley Encephalitis virus, Ross River virus, R0 model, temperature, vector- 19 borne disease 20 Running Title: Temperature & Ross River virus transmission Article type: Letter 21 Words in abstract: 147 Words in main text: 5000 Number of references: 94 22 Number of figures, tables and text boxes: 6 figures, no tables or text boxes 23 * Corresponding Author: Marta S. Shocket, [email protected], phone: 650-723-5923 1 bioRxiv preprint doi: https://doi.org/10.1101/286724; this version posted March 22, 2018. The copyright holder for this preprint (which was not certified by peer review) is the author/funder. All rights reserved. No reuse allowed without permission. 24 ABSTRACT 25 Temperature impacts the physiology of ectotherms, including vectors that transmit 26 disease. While thermal biology predicts nonlinear effects of temperature on vector and pathogen 27 traits that drive disease transmission, the empirical relationship between temperature and 28 transmission remains unknown for most vector-borne pathogens. We built a mechanistic model 29 to estimate the thermal response of Ross River virus, an important mosquito-borne pathogen of 30 humans in Australia, the Pacific Islands, and potentially emerging worldwide. Transmission 31 peaks at moderate temperatures (26.4°C) and declines to zero at low (17.0°C) and high (31.5°C) 32 temperatures. The model predicted broad patterns of disease across Australia. First, transmission 33 is year-round endemic in the tropics and sub-tropics but seasonal in temperate zones. Second, 34 nationwide human cases peak seasonally as predicted from population-weighted seasonal 35 temperatures. These results illustrate the importance of nonlinear, mechanistic models for 36 inferring the role of temperature in disease dynamics and predicting responses to climate change. 37 2 bioRxiv preprint doi: https://doi.org/10.1101/286724; this version posted March 22, 2018. The copyright holder for this preprint (which was not certified by peer review) is the author/funder. All rights reserved. No reuse allowed without permission. 38 INTRODUCTION 39 Temperature impacts mosquito-borne disease transmission via effects on the physiology 40 of mosquitoes and pathogens (Shocket et al. n.d.). Transmission requires that mosquitoes be 41 abundant, bite a host and ingest an infectious bloodmeal, survive long enough for pathogen 42 development and within-host migration (the extrinsic incubation period), and bite additional 43 hosts (Shocket et al. n.d.). All of these processes depend on temperature. Support for temperature 44 effects on mosquito-borne disease arises from mechanistic models based on thermal biology 45 (Mordecai et al. 2013, 2017; Liu-Helmersson et al. 2014; Wesolowski et al. 2015; Paull et al. 46 2017) and statistical models that analyze variation in climate and disease over time and space 47 (Werner et al. 2012; Stewart Ibarra & Lowe 2013; Siraj et al. 2015; Paull et al. 2017). However, 48 important knowledge gaps remain. First, variation in how transmission responds to temperature 49 across mosquito-borne diseases, and via what mechanisms, remains uncertain. Accordingly, the 50 types of data needed to characterize thermal responses of additional or emerging diseases are 51 unclear. Second, the impacts of temperature on transmission can appear idiosyncratic across 52 locations and studies (Hu et al. 2004; Gatton et al. 2005; Jacups et al. 2008a; Bi et al. 2009; 53 Werner et al. 2012; Koolhof et al. 2017), and inferring causality from field observations and 54 statistical approaches alone remains challenging. Thermal biology may provide an explanation 55 for this variation or a causal link. Filling these gaps is necessary to predict geographic, seasonal, 56 and interannual variation in transmission of mosquito-borne pathogens, especially as the climate 57 changes. Here, we address these gaps by building a model for temperature-dependent 58 transmission of Ross River virus. 59 Ross River virus (RRV) is a mosquito-transmitted alphavirus that causes the most 60 common mosquito-borne disease in Australia (1,500–9,500 human cases per year; Koolhof & 3 bioRxiv preprint doi: https://doi.org/10.1101/286724; this version posted March 22, 2018. The copyright holder for this preprint (which was not certified by peer review) is the author/funder. All rights reserved. No reuse allowed without permission. 61 Carver 2017). RRV is also endemic in Pacific Island nations, following a 1979-80 epidemic that 62 infected over 500,000 people (Klapsing et al. 2005; Aubry et al. 2015; Lau et al. 2017). RRV 63 infection causes acute fever, rash, and joint pain, which can become chronic and cause disability 64 (Harley et al. 2001). In some cases temperature predicts RRV cases (Gatton et al. 2005; Bi et al. 65 2009; Werner et al. 2012), while other cases it does not (Hu et al. 2004; Gatton et al. 2005). 66 Understanding RRV transmission ecology is critical because the virus is a likely candidate for 67 emergence worldwide (Flies et al. 2018). A mechanistic model for temperature-dependent RRV 68 transmission could help explain these disparate results and predict potential expansion. 69 Mechanistic models synthesize how environmental factors like temperature influence the 70 host and parasite traits that drive transmission. For ectotherms, these traits depend nonlinearly on 71 environmental temperature. Specifically, trait thermal responses are usually unimodal: they peak 72 at an intermediate temperature optimum and decline towards zero at lower and upper thermal 73 limits, all of which can vary across traits (Angilletta 2009; Dell et al. 2011; Mordecai et al. 2013, 74 2017). Mechanistic models combine the multiple, nonlinear thermal responses that shape 75 transmission (Rogers & Randolph 2006; Mordecai et al. 2013). One commonly-used measure of 76 disease spread is R0, the basic reproductive number (expected number of secondary cases from a 77 single case in a fully susceptible population). For mosquito-borne disease, R0 is a nonlinear 78 function of mosquito density, biting rate, vector competence (infectiousness given pathogen 79 exposure), and adult survival; pathogen extrinsic incubation period; and human recovery rate 80 (Dietz 1993). To understand the overall impact of temperature on transmission, we can 81 incorporate empirically-estimated trait thermal responses into an R0 model. Incorporating the full 82 suite of nonlinear trait responses often produces predictions that are drastically different than 83 models that assume linear or monotonic thermal responses or omit important temperature- 4 bioRxiv preprint doi: https://doi.org/10.1101/286724; this version posted March 22, 2018. The copyright holder for this preprint (which was not certified by peer review) is the author/funder. All rights reserved. No reuse allowed without permission. 84 dependent processes (Mordecai et al. 2013, 2017). Previous mechanistic models have predicted 85 different optimal temperatures for disease spread across pathogens and vector species: 25 ºC for 86 falciparum malaria and West Nile virus (Mordecai et al. 2013; Paull et al. 2017), and 29ºC and 87 26 ºC for dengue, chikungunya, and Zika viruses in Ae. aegypti and in Ae. albopictus, 88 respectively (Liu-Helmersson et al. 2014; Wesolowski et al. 2015; Mordecai et al. 2017). 89 In this paper, we build the first mechanistic model for temperature-dependent 90 transmission of RRV and ask whether temperature explains seasonal and geographic patterns of 91 disease spread. We use data from laboratory experiments with the two most important and well- 92 studied vector species (Culex annulirostris and Aedes vigilax) to parameterize the model with 93 unimodal thermal responses. We then use sensitivity and uncertainty analyses to determine 94 which traits drive the relationship between temperature and transmission potential and identify 95 key data gaps. Finally, we illustrate how temperature currently shapes patterns of disease across 96 Australia. The model correctly predicts that RRV disease should be year-round endemic in 97 tropical, northern Australia and seasonally epidemic in temperate, southern Australia. It also 98 accurately predicts the seasonality of human cases nationally. Thus,
Details
-
File Typepdf
-
Upload Time-
-
Content LanguagesEnglish
-
Upload UserAnonymous/Not logged-in
-
File Pages36 Page
-
File Size-