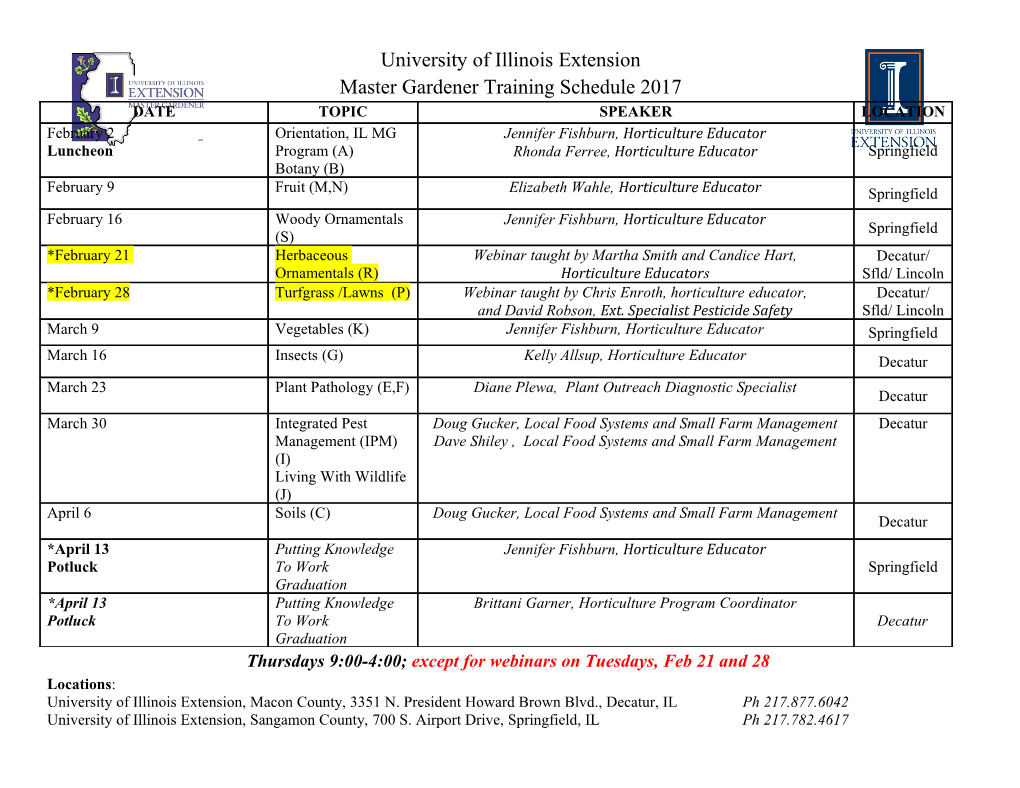
PUBLICATIONS Journal of Geophysical Research: Solid Earth RESEARCH ARTICLE The role of pyroxenite in basalt genesis: Melt-PX, a melting 10.1002/2015JB012762 parameterization for mantle pyroxenites between Key Points: 0.9 and 5GPa • Melt-PX predicts the melting behavior of pyroxenites as a function of P, T, Sarah Lambart1,2, Michael B. Baker1, and Edward M. Stolper1 and X • > At P ~3.5 GPa, a large fraction 1Division of Geological and Planetary Sciences, California Institute of Technology, Pasadena, California, USA, 2Department (>20%) of natural pyroxenites have near-solidus temperatures greater of Earth and Planetary Sciences, University of California, Davis, California, USA than those of fertile peridotite • Crustal thickness depends on the mass fraction and composition of Abstract Geochemical and isotopic data suggest that the source regions of oceanic basalts may contain pyroxenite in the mantle as well as pyroxenite in addition to peridotite. In order to incorporate the wide range of compositions and melting mantle potential temperature behaviors of pyroxenites into mantle melting models, we have developed a new parameterization, Melt-PX, which predicts near-solidus temperatures and extents of melting as a function of temperature and pressure Supporting Information: for mantle pyroxenites. We used 183 high-pressure experiments (25 compositions; 0.9–5 GPa; 1150–1675°C) • Supporting Information S1 • Table S1 to constrain a model of melt fraction versus temperature from 5% melting up to the disappearance of • Melt-PX spreadsheet clinopyroxene for pyroxenites as a function of pressure, temperature, and bulk composition. When applied to the global set of experimental data, our model reproduces the experimental F values with a standard error of Correspondence to: estimate of 13% absolute; temperatures at which the pyroxenite is 5% molten are reproduced with a S. Lambart, [email protected] standard error of estimate of 30°C over a temperature range of ~500°C and a pressure range of ~4 GPa. In conjunction with parameterizations of peridotite melting, Melt-PX can be used to model the partial melting of multilithologic mantle sources—including the effects of varying the composition and the modal Citation: Lambart, S., M. B. Baker, and E. M. Stolper proportion of pyroxenite in such source regions. Examples of such applications include calculations of (2016), The role of pyroxenite in isentropic decompression melting of a mixed peridotite + pyroxenite mantle; these show that although the basalt genesis: Melt-PX, a melting potential temperature of the upwelling mantle plays an important role in defining the extent of magma parameterization for mantle pyroxenites between 0.9 and 5 GPa, J. Geophys. Res. production, the composition and mass fraction of the pyroxenite also exert strong controls. Solid Earth, 121, 5708–5735, doi:10.1002/ 2015JB012762. 1. Introduction Received 19 DEC 2015 Melting of mantle sources with multiple lithologies, each with its own melting behavior and chemical and Accepted 14 JUL 2016 Accepted article online 16 JUL 2016 isotopic composition, is believed to be an important factor in producing the range of magma compositions Published online 18 AUG 2016 observed in individual igneous provinces [Hofmann, 2007; Kogiso et al., 2004a; Lambart et al., 2013, and references therein]. The notion that pyroxenites—olivine-poor lithologies dominated by pyroxene under upper mantle conditions—play a significant role in basalt genesis is supported by various geochemical and petrological observations: 1. Although it is widely debated as to when plate tectonics began (see Arndt [2013] and Korenaga [2013] for reviews), oceanic crust (which converts to pyroxene-rich rocks under mantle conditions) has likely been subducted back into the mantle over the last several gigayears and has been suggested as a reservoir in the mantle for certain incompatible trace elements [e.g., Hauri and Hart, 1997; Kamber and Collerson, 2000; McDonough, 1991; Rudnick et al., 2000; Sun et al., 2011]. Other processes such as lithospheric dela- mination [e.g., Elkins-Tanton, 2005; Kay and Mahlburg Kay, 1993; Lee, 2014], mantle wedge metasomatism [e.g., Kelemen et al., 1998; Rapp et al., 1999; Straub et al., 2008; Yaxley and Green, 1998], and emplacement of magmas and metasomatism in the lithosphere [e.g., Humphreys and Niu, 2009; Niu, 2008; Pilet et al., 2005, 2008] can also introduce pyroxene-rich lithologies into the mantle. 2. Pyroxenites are ubiquitous in orogenic massifs and ophiolites [Bodinier and Godard, 2003] and are also ©2016. The Authors. sampled as mantle xenoliths [Pearson et al., 2003]. Based on the proportions of pyroxenites in orogenic This is an open access article under the massifs, pyroxenites may constitute 2 to 5% of the Earth’s upper mantle and can locally reach up to 10% terms of the Creative Commons (e.g., in Beni Bousera [Pearson and Nixon, 1996]). Such occurrences of pyroxene-rich rocks in the upper Attribution-NonCommercial-NoDerivs License, which permits use and distri- mantle inspired the “marble cake model” of Allègre and Turcotte [1986]. bution in any medium, provided the 3. High-pressure melting experiments on pyroxene-rich lithologies [e.g., Green and Ringwood, 1967; original work is properly cited, the use is Kornprobst, 1970; Lambart et al., 2009a; Pertermann and Hirschmann, 2003a; Yasuda et al., 1994; Yaxley non-commercial and no modifications or adaptations are made. and Green, 1998] demonstrate that at the same pressure, many pyroxenites have narrower melting LAMBART ET AL. MELT-PX: PYROXENITE MELTING MODEL 5708 Journal of Geophysical Research: Solid Earth 10.1002/2015JB012762 intervals and lower solidus temperatures than peridotites. Such pyroxenites entrained in an adiabatically upwelling mantle consisting largely of peridotite would contribute a greater fraction of liquid to the aggregated mantle partial melt than would be inferred from their relatively low mass fraction in the region undergoing melting [Hirschmann and Stolper, 1996; Phipps Morgan, 2001; Sleep, 1984; Stolper and Asimow, 2007]. For example, Hirschmann and Stolper [1996] estimated that a mantle containing 5% pyrox- enite and 95% peridotite could generate a magma with ~15% pyroxenite-derived melt, and Lambart et al. [2009a] suggested that the proportion of pyroxenite-derived melt in mid-ocean ridge basalts (MORBs) may reach up to 40% along some ridge segments. 4. Numerous observations and models suggest that partial melts from pyroxene-rich lithologies are compo- nents in erupted basaltic magmas [e.g., Day et al., 2009; Hauri, 1996; Herzberg, 2006, 2011; Hirschmann and Stolper, 1996; Hofmann and White, 1982; Lassiter et al., 2000; Prinzhofer et al., 1989; Salters and Dick, 2002; Sigmarsson et al., 1998; Sobolev et al., 2005, 2008]. For example, Hf isotopic compositions, rare earth element distributions, and (230Th)/(238U) disequilibria observed in MORBs have been used to suggest the presence of residual garnet in their mantle sources [e.g., Beattie, 1993; Kay and Gast, 1973]. Although melting in the garnet stability field of peridotites (at pressures > 2.5 GPa) [Kinzler, 1997] is expected to result in amounts of melting [Iwamori et al., 1995; Robinson and Wood, 1998; Shen and Forsyth, 1995] that would lead to crustal thicknesses substantially greater than that observed for normal oceanic crust (7.1 ± 0.8 km [White et al., 1992]), melt contributions from pyroxenite, where garnet coexist- ing with liquid extends to lower pressures (down to ~1.7 GPa) [Kogiso et al., 2004a], might explain both the “garnet signature” of MORBs and the observed crustal thicknesses [Hirschmann and Stolper, 1996]. Likewise, the HIMU isotopic compositions of oceanic island basalts (OIBs) have been attributed to the presence in their source regions of clinopyroxene-rich metasomatic veins from subducted lithosphere [Pilet et al., 2011] or of carbonated eclogite [Dasgupta et al., 2010]. In another example, Sobolev et al. [2007] used olivine phenocryst compositions (e.g., Ni and Mn) to suggest that mantle-derived magmas at mid-ocean ridges, oceans islands, and large igneous provinces are mixtures of melts of peridotite and pyroxenite, where the component derived from pyroxenite melting can vary from 10 to 100% of the mass of the basalt. Finally, silica-poor and iron-rich examples of MORBs [Lambart et al., 2009a, 2013] and the generally high CaO/Al2O3 and FeO and TiO2 contents of OIBs relative to MORBs [Hirschmann et al., 2003; Kogiso et al., 2003; Prytulak and Elliott, 2007] have also been attributed to the melting of a pyroxenite component in their mantle source regions. Given the potential for pyroxene-rich lithologies to contribute to the observed compositional and isotopic variability of basaltic magmas and the many cases in which partial melts of pyroxenites have been invoked to explain the petrogenesis of specific basalts, it is not surprising that there is a large and grow- ing body of literature that has focused on the melting of pyroxenite-bearing mantle [e.g., Allègre and Turcotte, 1986; Brown and Lesher, 2014; Hirschmann and Stolper, 1996; Ito and Mahoney, 2005; Kimura and Kawabata, 2015; Lassiter et al., 2000; Pertermann and Hirschmann, 2003a; Phipps Morgan, 2001; Prinzhofer et al., 1989; Sleep, 1984; Stolper and Asimow, 2007]. However, because natural pyroxenites span a wide compositional range with a correspondingly wide range of solidus temperatures and phase relations [Kogiso et al., 2004a, and references therein], modeling their contribution to melt production
Details
-
File Typepdf
-
Upload Time-
-
Content LanguagesEnglish
-
Upload UserAnonymous/Not logged-in
-
File Pages28 Page
-
File Size-