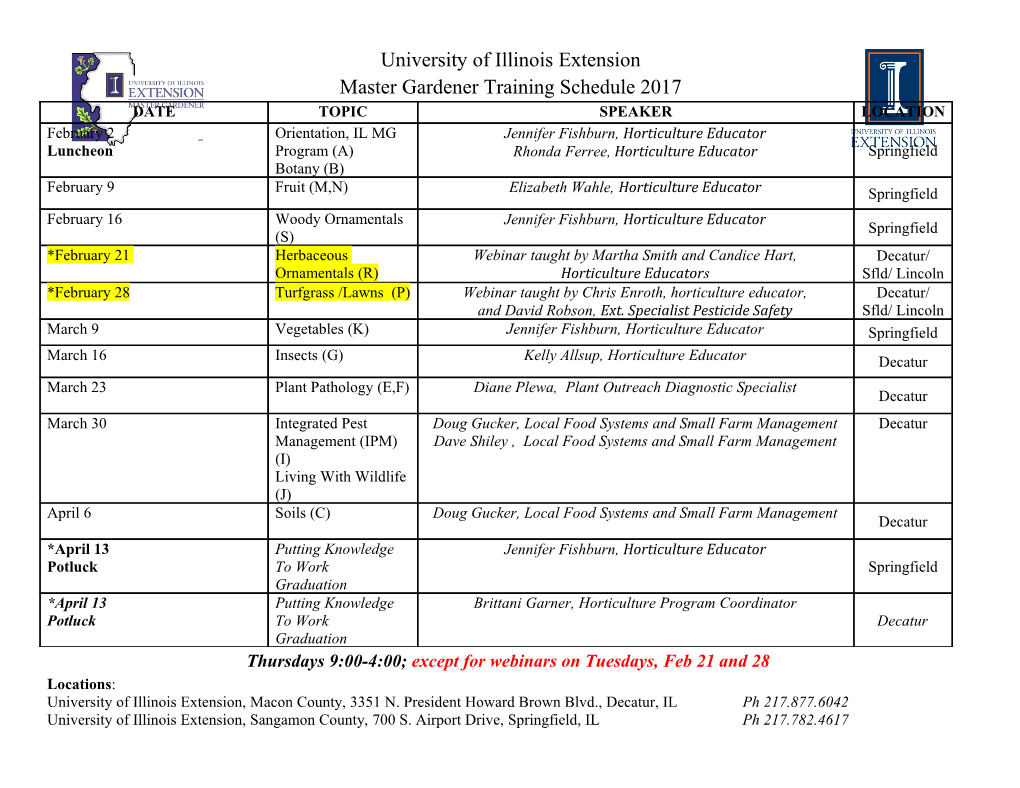
Sorbonne Université ED 388 - Ecole doctorale de Chimie Physique et de Chimie Analytique de Paris Centre Représentation d’une huile dans des simulations mésoscopiques pour des applications EOR par David Steinmetz Thèse de doctorat de Chimie Dirigée par Carlos Nieto-Draghi Présentée et soutenue publiquement le 19/10/2018 Devant un jury composé de : Prof. Malfreyt Patrice Rapporteur Prof. Lisal Martin Rapporteur Prof. Jardat Marie Examinateur Prof. Carbone Paola Examinateur Dr. Lemarchand Claire Examinateur Dr. Nieto-Draghi Carlos Directeur de thèse Dr. Creton Benoît Examinateur Sorbonne University ED 388 - Ecole doctorale de Chimie Physique et de Chimie Analytique de Paris Centre Representation of an oil in mesoscopic simulations for EOR applications by David Steinmetz Chemistry Ph.D. Thesis Directed by Carlos Nieto-Draghi Presented and defended publicly on 19 October 2018 In front of jury: Prof. Malfreyt Patrice Reviewer Prof. Lisal Martin Reviewer Prof. Jardat Marie Examiner Prof. Carbone Paola Examiner Dr. Lemarchand Claire Examiner Dr. Nieto-Draghi Carlos Thesis supervisor Dr. Creton Benoît Examiner Acknowledgement Firstly, I would like to thank Ms. Ruffier-Meray, head of the Direction Chimie et Physico-Chimie Appliquées, and Mr. Mougin, head of the Departement Thermodynamique et Modélisation Moléculaire, for welcoming me at IFP Energies nouvelles and allowing me to do this work in excellent conditions. I would like to sincerely thank my thesis supervisor, Carlos Nieto-Draghi, for the continuous support of my Ph.D study and research. I have been extremely lucky to have a supervisor who cared so much about my work. I appreciated his great efforts to explain things clearly and simply. I would like to express my deep gratitude to my supervisor Benoît Creton. This work would not have been possible without his guidance, support and encouragement. Under his guidance, I successfully overcame many difficulties and leaned a lot. Véronique Lachet gave me invaluable help during my thesis and I am grateful to for her advices and valuable comments. I also wish to express my gratitude to Bernard Rousseau for his valuable advice, constructive criticism and his extensive discussions around my work. Most of the results described in this thesis would not have been obtained without the involvement of Jan Verstraete. I would like to thank him for sharing his expertise in petroleum field. I am also deeply thankful to Rafael Lugo for fruitful discussions and for allowing me to understand some aspects of thermodynamics. A very special thanks goes out to all the Ph.D students and colleagues: Saifuddin, Germain, Mona, Michel, Anand, Xavier and Sébastien for providing a stimulating and fun environment and for keeping me motivated throughout my thesis. I take this opportunity to express my gratitude to all the people of the department for the warm welcome. 1 Table of contents ACKNOWLEDGEMENT 1 TABLE OF CONTENTS 2 INTRODUCTION 5 CHAPTER 1. SETTING UP STAGE 8 1.1 INTRODUCTION 8 1.2 CRUDE OIL AND PETROLEUM FRACTIONS 8 1.2.1 FORMATION OF PETROLEUM 8 1.2.2 DESCRIPTION OF PETROLEUM 9 1.2.3 DESCRIPTION OF PETROLEUM FRACTIONS 10 1.3 MOLECULAR COMPOSITION OF CRUDE OIL 12 1.3.1 HYDROCARBONS 12 1.3.2 OTHER CRUDE OIL COMPONENTS 14 1.4 CRUDE OIL EXTRACTION 17 1.4.1 CRUDE OIL EXTRACTION METHODS 17 1.4.2 RECOVERY OF RESIDUAL OIL USING EOR TECHNIQUES 18 1.5 CONCLUSIONS 21 CHAPTER 2. CHARACTERIZATION OF CRUDE OIL 22 2.1 INTRODUCTION 22 2.2 PROPERTIES OF CRUDE OIL: EXPERIMENTAL METHODS 23 2.2.1 ELEMENTAL ANALYSIS 23 2.2.2 DENSITY AND SPECIFIC GRAVITY 24 2.2.3 MOLECULAR WEIGHT 25 2.2.4 BOILING TEMPERATURE AND DISTILLATION CURVES 25 2.2.5 CHEMICAL FAMILIES 27 2.2.6 INTERFACIAL TENSION 28 2.3 STATE OF THE ART FOR THE REPRESENTATION OF CRUDE OIL 30 2.3.1 FRACTIONATION APPROACHES 30 2.3.2 LUMPING METHODS 32 2.3.3 MOLECULAR RECONSTRUCTION 34 2.4 CONCLUSIONS 38 CHAPTER 3. COARSE-GRAINED SIMULATION METHODS 40 3.1 INTRODUCTION 40 3.2 DISSIPATIVE PARTICLES DYNAMICS (DPD) 42 3.2.1 CONSERVATIVE FORCE 42 3.2.2 INTRAMOLECULAR FORCE 43 3.2.3 DISSIPATIVE AND RANDOM FORCES 44 3.2.4 FLUCTUATION-DISSIPATION THEOREM 45 2 3.2.5 TIME INTEGRATION SCHEMES 46 3.3 COARSE-GRAINED-MONTE CARLO SIMULATION (CG-MC) 47 3.3.1 PRINCIPLE AND METROPOLIS ALGORITHM 47 3.3.2 STATISTICAL ENSEMBLES 48 3.3.3 MONTE CARLO MOVES 50 3.4 COARSE-GRAINED SIMULATIONS 53 3.4.1 COARSE-GRAINED MODEL 53 3.4.2 SIMULATIONS BOXES AND BOUNDARY CONDITIONS 54 3.4.3 INTERFACIAL TENSION CALCULATION 55 3.4.4 COARSE-GRAINED UNITS 59 3.5 CONCLUSIONS 60 CHAPTER 4. PARAMETERIZATION OF LIQUID-LIQUID TERNARY MIXTURES 61 4.1 INTRODUCTION 61 4.2 STATE OF THE ART OF PARAMETERIZATION OF COARSE-GRAINED SIMULATIONS 64 4.3 NEW PARAMETERIZATION APPROACH FOR LIQUID-LIQUID EQUILIBRIUM OF TERNARY SYSTEMS: METHODOLOGY AND COMPOSITIONAL DETAILS 68 4.3.1 PARAMETERIZATION OF INTERACTIONS 68 4.3.2 STATISTICAL ENSEMBLES – METHODOLOGY TO COMPUTE IFT 72 4.3.3 SYSTEMS STUDIED 75 4.4 RESULTS 78 4.4.1 COMPOSITION DEPENDENCE OF THE FLORY-HUGGINS INTERACTION PARAMETERS 78 4.4.2 LIQUID-LIQUID EQUILIBRIUM (LLE) 80 4.4.3 INTERFACE COMPOSITIONS 86 4.4.4 INTERFACIAL TENSION 87 4.5 THERMODYNAMIC MODELS FOR PREDICTION OF LIQUID-LIQUID EQUILIBRIA 93 4.5.1 PREDICTION OF THE COMPOSITION OF LIQUID-LIQUID EQUILIBRIA 93 4.5.2 PREDICTION OF INTERACTION PARAMETERS USING A THERMODYNAMIC MODEL 98 4.5.3 TRANSFERABILITY OF INTERACTION PARAMETERS FOR ALKANES 102 4.6 CONCLUSIONS 104 CHAPTER 5. REPRESENTATION AND COARSE-GRAINED SIMULATIONS OF CRUDE OILS 106 5.1 INTRODUCTION 106 5.2 THEORETICAL BACKGROUND FOR CRUDE OIL MOLECULAR REPRESENTATION 108 5.2.1 REPRESENTATION OF THE LIGHT FRACTION (C20-): THE LUMPED ALGORITHM 108 5.2.2 REPRESENTATION OF HEAVY FRACTIONS (C20+) 109 5.3 RESULTS 118 5.3.1 MOLECULAR MODEL OF CRUDE OIL 118 5.3.2 COARSE-GRAINED MODEL OF CRUDE OIL 126 5.3.3 PARAMETERIZATION OF INTERACTIONS FOR DPD SIMULATIONS 128 5.4 RESULTS OF DPD SIMULATIONS OF CRUDE OIL 131 5.5 CONCLUSIONS 134 GENERAL CONCLUSION 136 BIBLIOGRAPHY 140 3 APPENDICES 150 APPENDICES – CHAPTER 4 150 APPENDICES – CHAPTER 5 182 LIST OF FIGURES 192 LIST OF TABLES 197 4 Introduction The organic origin of petroleum is the most widely accepted, and from this hypothesis oil and gas result from the slow decomposition of plants and animals over millions of years. Its high energy density makes it an ideal fuel for transportation (cars, trucks, airplanes, etc.). Nowadays, it is an irreplaceable raw material used by the petrochemical industry to produce polymers, plastics, paints, dyes, cosmetics, etc. Some fractions of petroleum are also used as a fuel in domestic heating and as a source of heat in industry [1]. Despite the known impact on environment, petroleum is the most important source of energy, and fossil energy represents about 80% of the total energy consumption in the world1. Crude oil is found in Earth’s subsurface areas called reservoirs. Its extraction usually takes place into three phases. Primary oil recovery technique relies on the existing natural difference of pressure between the reservoir and surface. The recovery rate is low and generally around 5% OOIP (Original Oil in Place) for heavy oils and can reach 25% OOIP for light oils [2]. Secondary recovery allows, on average, to increase the recovery rate to 30% OOIP by maintaining a pressure in the reservoir by injection of water or gas [2]. However, a large part of the oil remains stuck in the pores of the rock due to capillary forces [3]. Tertiary recovery methods called Enhanced Oil Recovery (EOR) have been developed in order to increase significantly the quantities of oil that can be extracted from a reservoir. EOR methods aim to affect the properties of mobility and wettability of the oil to facilitate its displacement to production wells. Several EOR processes exist to increase the mobility of oil by modifying either (i) the temperature of the reservoir by the injection of hot water or steam (ii) the miscibility of the oil by the addition of a phase of hydrocarbons (iii) or the interfacial tension between oil and water by the introduction of an ASP (Alkaline/Surfactant/Polymer) formulation. This latter process is called chemical EOR (cEOR). Improving cEOR techniques is one of the main research focuses of “The EOR Alliance” formed by IFPEN, Solvay, and Beicip Franlab companies. The approach consists of determining the best ASP combinations to mobilize the oil trapped in the reservoir by acting on capillary forces. To reduce capillary forces, viscosity of the fluid can be increased and/or the interfacial tension (IFT) between oil and water reduced. The ASP formulation aims at 1 bp.com/statisticalreview 5 reaching an ultralow IFT for the brine/surfactant/crude oil systems. However, the difficulty of the formulation design stands in the fact that each oil reservoir is unique and they differ from each other by their composition, salinity, pressure, and temperature condition. A specific S/SP/ASP formulation for a given reservoir is necessary. Because of complex involved phenomena, identification and selection of relevant surfactants is challenging, and it requires a large number of trial-and-error tests. Modelling tools such as molecular simulations are adapted to improve the efficiency of such a process by providing information about phenomena occurring at the molecular level and at the interface [4]. Molecular simulation of petroleum requires establishing the molecular representation of the fluid. However, petroleum is a mixture of molecules so complex that even the most efficient analytical techniques do not identify molecules one by one.
Details
-
File Typepdf
-
Upload Time-
-
Content LanguagesEnglish
-
Upload UserAnonymous/Not logged-in
-
File Pages204 Page
-
File Size-