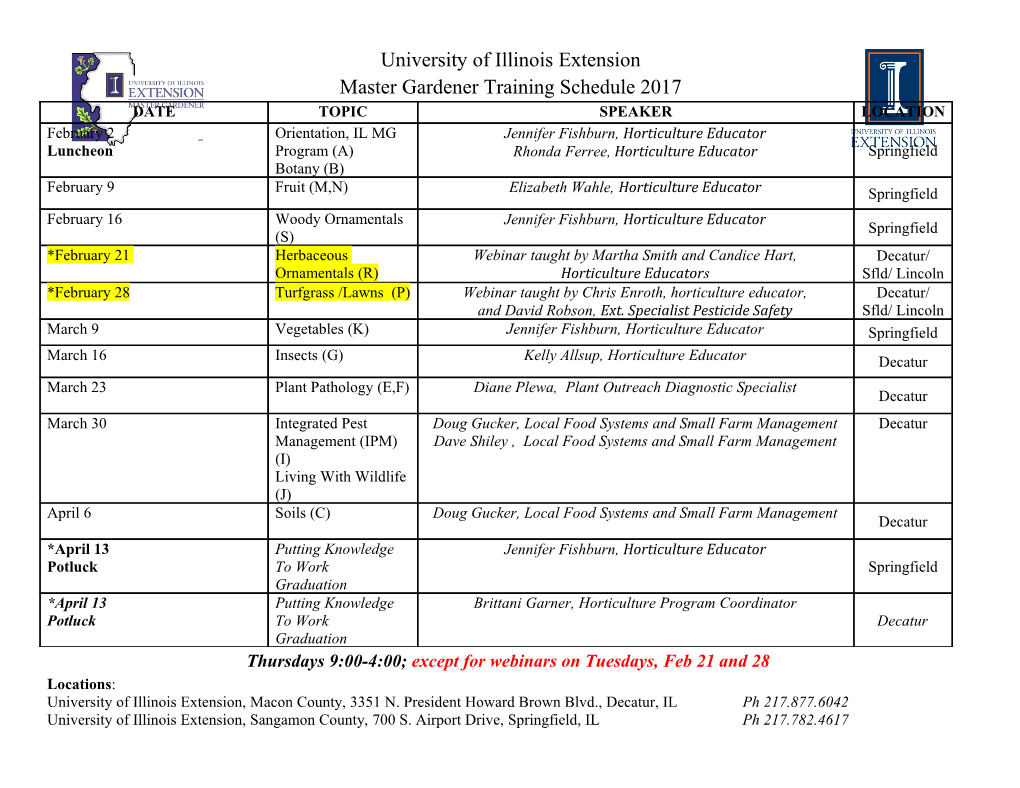
U N I V E R S I D A D D E C O N C E P C I Ó N DEPARTAMENTO DE CIENCIAS DE LA TIERRA 10° CONGRESO GEOLÓGICO CHILENO 2003 SEARCHING FOR CAUSES OF ANDEAN SEGMENTATION (15°-47°S): INTERPRETATION OF ELASTIC THICKNESS AND ISOTOPIC DATA, PLUS A PRELIMINARY 3D DENSITY MODEL TASSARA, A., GÖTZE, H-J., LUCASSEN, F. Institut für Geologische Wissenschaften, Freie Universität Berlin, Malteserstrasse 74-100, D-12249 Berlin, Germany [email protected] 1. INTRODUCTION The Andean Cordillera is a continuous feature along the western margin of South America that has been constructed by a simple common process of ocean-continent collision. However, at a continental scale the Andes show a remarkable along-strike segmentation of topography, volcanism, tectonics, geological history, subduction geometry and deep structure (e.g. Jordan et al., 1983; Mpodozis & Ramos, 1989; Cahill & Isacks, 1992; Kley et al., 1999; Tassara & Yañez, in press). The central (15°-33.5°S) and southern (33.5°-47°S) segments of the Andes constitute an excellent area to study the causes of this segmentation. In response to a convergence vector that does not exhibit spatial variations in azimuth and speed, the Central Andes developed a huge mountain belt that starkly contrasts with the narrow and small Southern Andes (fig. 1). This fact, added to the persistency of the Andean segments throughout hundred of millions years (e.g. Mpodozis & Ramos, 1989), suggests that this segmentation is mainly controlled by inherited rheological variations along the continental plate. Here we analyse this hypothesis using two different approaches. First, a quantitative interpretation of elastic thickness estimates in the framework of a brittle-elasto-ductile rheology is presented. This exercise, constrained by isotopic data, indicates that along-strike variations in crustal composition are the main factor controlling the rigidity differences between the Central and Southern Andes. Secondly, the results of a preliminary three-dimensional (3D) density model between 15°-47°S are presented. This model is focused on the identification of first-order along- strike variations of Andean crustal structure (geometry and composition). 2. DESCRIPTION OF THE ALONG-STRIKE SEGMENTATION OF THE ANDES (15°- 47°S) The position of oceanic ridges and the morphotectonic units forming the continental margin between 15°S and 47°S are depicted in figure 1. This figure also presents proposed boundaries and nomenclature for the segmentation along the Andean margin (after Tassara & Yañez, in press). The boundaries of the Central and Southern Andes correspond to the intersection of oceanic ridges with the Peru-Chile trench. Along the northern and southern boundaries of the Central Andes, the intermediate depth (100-200 km) dip angle of the subducted Todas las contribuciones fueron proporcionados directamente por los autores y su contenido es de su exclusiva responsabilidad. slab changes abruptly from “flat” (<10°) to “normal” (25-30°) (e.g. Cahill & Isacks, 1992). Fig. 1. Morphotectonic units of western South American between 15°S and 47°S and oceanic ridges of the Nazca plate. Boundaries and nomenclature for the Andean segmentation after Tassara & Yañez (in press). Abbreviations in bold emphasize morphotectonic units commented in the text: ssa Sierras Subandinas, ap Altiplano, wc Western Cordillera, pn Puna, sbs Santa Barbara System, cc Coastal Cordillera, fc Frontal Cordillera, sp Sierras Panpeanas, pc Principal Cordillera, pgc Patagonian Cordillera. Thin lines denote 50 km contour lines of the subducted slab (Cahill & Isacks, 1992). Triangles are active volcanoes of the central (grey, CVZ) and southern (white, SVZ) volcanic zones. Both the Central and Southern Andes can be subdivided into second-order segments. We describe here some aspects of this second-order segmentation to be discussed in the next sections. The width of the orogen decreases along the Central Andes from more than 600 km at the Altiplano Plateau to less than 200 km at the Frontal Cordillera segment. This narrowing correlates with a decrease in Neogene crustal shortening and a systematic change of the active foreland deformation styles: thin skinned at the Sierras Subandinas, thick skinned at the Santa Barbara System, and basement uplift at the Sierras Pampeanas (e.g. Kley et al., 1999). South of 24°S, the subducted slab shows a sub-horizontal zone below 100 km depth that reaches a maximum width of ~450 km at 33°-34°S (e.g. Cahill & Isacks, 1992). The absence of asthenospheric mantle below the Frontal Cordilleran lithosphere precludes the occurrence of a magmatic arc. Thus, the Central Volcanic Zone (CVZ) of the Andes is restricted to the Altiplano and Puna segments. The CVZ is composed of andesitic-dacitic calcalkaline stratovolcanoes located on top of the Western Cordillera and bi-modal volcanic products deposited on the Altiplano-Puna (e.g. Allmendinger et al., 1997). The magmas of the CVZ have assimilated a relatively high degree of felsic crust during their evolution (e.g. Kay et al., 1999). South of 33.5°S, elevations higher than ~4000 m decrease gradually along the Principal Cordillera segment to less than 2000 m at the Patagonian Cordillera segment. This decrease also correlates with north-south variations in the Neogene tectonic style: thick-skinned foreland tectonics linked with trench-oblique structures at the Principal Cordillera segment, and trench- parallel strike-slip deformation along the Liquiñe-Ofqui Fault Zone (LOFZ) at the Patagonian Cordillera segment (e.g. Folguera et al., 2002). South of 34°S the subducted slab has a normal intermediate dip angle. This drives the mantle magmagenesis linked to the presence of the Southern Volcanic Zone (SVZ) of the Andes. The northern part of the SVZ, on top of the Principal Cordillera, is composed of andesitic calcalkaline stratovolcanoes with a crustal magmatic component decreasing toward the south (e.g. Hildret & Moorbarth, 1988). The southern SVZ builds the western-central part of the Patagonian Cordillera and is genetically related to the LOFZ (e.g. Lopez-Escobar et al., 1995). This volcanic chain is composed of andesitic and basaltic stratovolcanoes of calcalkaline to tholeitic affinities and several minor eruptive centres. 3. ISOTOPIC DATA, ELASTIC THICKNESS AND ANDEAN SEGMENTATION 3.1. ISOTOPIC CHARACTERIZATION OF THE ANDEAN CRUST Figure 2 summarises new unpublished and published Sr, Nd and Pb isotopic data. Isotopic compositions of typical magmatic, metamorphic, and sedimentary rocks constrain the crustal make up in the Central Andes between 18° and 28°S and the Southern Andes between 36° and 40°S. Fig. 2. Isotopic composition of Palaeozoic magmatic and metamorphic rocks (Palaeozoic crust), Meso-Cenozoic magmatic rocks and Neogene volcanic rocks from regions in the Central (18°-28°S, red symbols) and Southern (36°- 40°S, blue symbols) Andes. Data of pre-Neogene rocks are corrected for in-situ decay. Data sources: Patagonian Batholith, Pankhurst et al. (1999); Central Andes Jurassic – early Cretaceous, Lucassen et al. (2002); SVZ Cenozoic volcanic rocks, Hildreth and Moorbath (1988); Southern Andes pre-Neogene rocks, unpublished data of Lucassen (pers. comm.); All other data see Lucassen et al. (2001) and ref. therein. From diagrams of figure 2, we observe the following points: 1. Isotopic composition of sampled Palaeozoic basement is very similar at both areas (fig. 3c-d). These Palaeozoic rocks are predominantly felsic in composition and represent the common crust of the Andean continental margin. 2. The felsic Palaeozoic crust in both areas has been compositionally modified by Meso- Cenozoic mafic-intermediate arc magmatism. The juvenile arc magmas came from a depleted mantle source (fig. 3a-b-e). 3. In the Central Andes, this process took place during the evolution of the nearly stationary Jurassic – early Cretaceous arc along the Coastal Cordillera. Later eastward migration of magmatism had only minor effect on the composition of the common Palaeozoic crust. Isotopic composition of the CVZ volcanic rocks shows a considerable contribution of the Palaeozoic crust (fig. 3b-e). 4. In the southern studied area, a continuous addition of juvenile magmas since ca. 200 My has occurred in a nearly stationary magmatic arc located in the Patagonian Cordillera. The new Meso-Cenozoic mafic-intermediate crust (Northpatagonian batholith) has largely an isotopic composition of depleted mantle (fig. 3a-b-e). Volcanic rocks of the SVZ are isotopically indistinguishable from the extended Meso-Cenozoic intrusions (fig. 3a). 5. In summary, along the axis of the present Andean magmatic arc, the bulk crustal composition revealed by isotopic data, changes from dominant felsic in the Central Andes (Palaeozoic crust) to mafic-intermediate in the Southern Andes (Northpatagonian batholith). 3.2. ELASTIC THICKNESS ESTIMATES The elastic thickness Te controls the flexural rigidity of the lithosphere. Thus, Te is a relevant parameter describing the long-term mechanical behaviour of the lithosphere (e.g. Burov & Diament, 1995). Tassara (1997; in press) and Tassara & Yañez (1996; in press, hereafter T&Y) present Te estimates for 15 profiles perpendicular to the trench axis along the Andean margin between 15° and 47°S. Forward modelling of the Bouguer anomaly was performed along each profile with the assumption that the continental lithosphere is a thin elastic plate of variable thickness (Te) deflected under topographic loads (full description in Tassara, 1997). Fig. 3. After Tassara & Yañez (in press). a) Elastic thickness estimates across each modelled profile showing differences in Te throughout variations in colour and size. Moho depth Zm map was interpolated from values obtained after inversion of the modelled Bouguer anomaly for each profile. b) North-South profile A-A´ illustrates along- strike variations of elastic and crustal thickness. Figure 3a shows the final Te profiles of T&Y on a Moho depth (Zm) map interpolated from the inversion of the modelled Bouguer anomaly. The elastic thickness is low along the orogen axis (< 45 km) and high in the rigid forearc and foreland flanks (maximum Te ~70 km).
Details
-
File Typepdf
-
Upload Time-
-
Content LanguagesEnglish
-
Upload UserAnonymous/Not logged-in
-
File Pages10 Page
-
File Size-