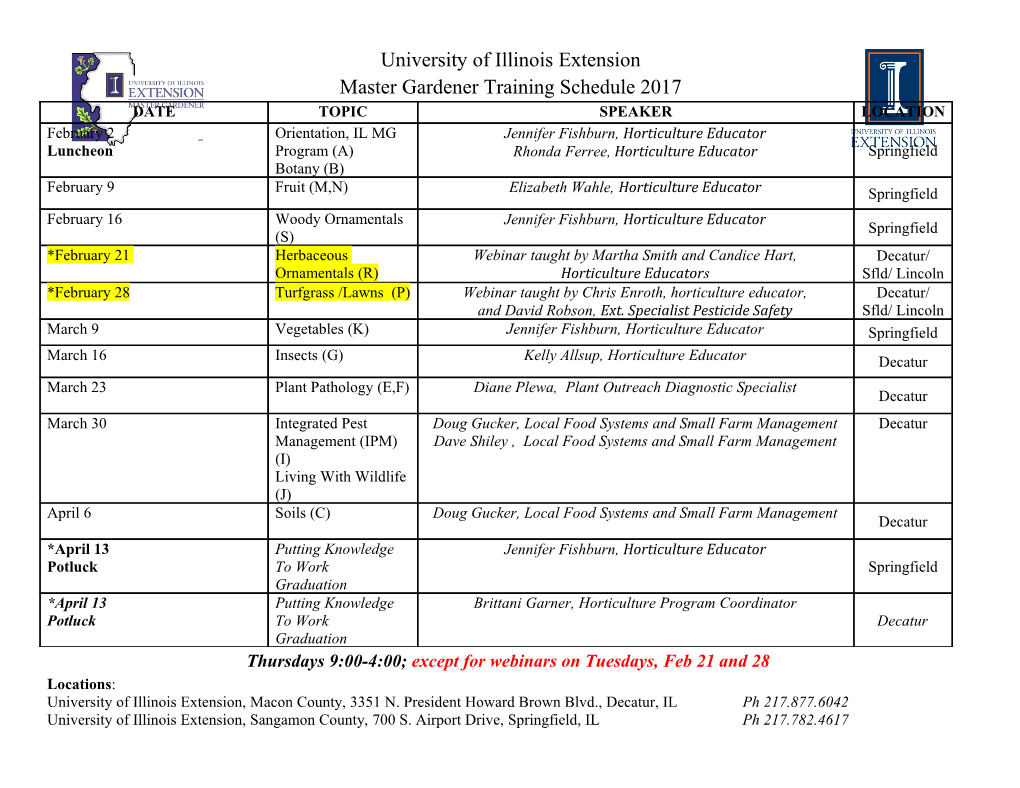
Tall Pinus luzmariae trees with genes from P. herrerae Christian Wehenkel1, Samantha del Rocío Mariscal-Lucero1,2, M. Socorro González-Elizondo3, Víctor A. Aguirre-Galindo1, Matthias Fladung4 and Carlos A. López-Sánchez5 1 Instituto de Silvicultura e Industria de la Madera, Universidad Juárez del Estado de Durango, Durango, Mexico 2 Instituto Tecnológico del Valle del Guadiana, Tecnológico Nacional de México, Durango, Mexico 3 CIIDIR Durango, Instituto Politécnico Nacional, Durango, Mexico 4 Thünen-Institute of Forest Genetics, Grosshansdorf, Germany 5 Department of Organisms and Systems Biology, University of Oviedo, Polytechnic School of Mieres, Asturias, Spain ABSTRACT Context. Pinus herrerae and P. luzmariae are endemic to western Mexico, where they cover an area of more than 1 million hectares. Pinus herrerae is also cultivated in field trials in South Africa and South America, because of its considerable economic importance as a source of timber and resin. Seed quality, afforestation success and desirable traits may all be influenced by the presence of hybrid trees in seed stands. Aims. We aimed to determine the degree of hybridization between P. herrerae and P. luzmariae in seed stands of each species located in the Sierra Madre Occidental, Durango, Mexico. Methods. AFLP molecular markers from samples of 171 trees across five populations were analyzed with STRUCTURE and NewHybrids software to determine the degree of introgressive hybridization. The accuracy of STRUCTURE and NewHybrids in detecting hybrids was quantified using the software Hybridlab 1.0. Morphological analysis of 131 samples from two populations of P. herrerae and two populations of P. luzmariae was also conducted by Random Forest classification. The data were compared Submitted 30 July 2019 by Principal Coordinate Analysis (PCoA) in GenAlex 6.501. Accepted 27 January 2020 Results. Hybridization between Pinus herrerae and P. luzmariae was observed in all seed Published 26 February 2020 stands under study and resulted in enhancement of desirable silvicultural traits in the Corresponding author latter species. In P. luzmariae, only about 16% molecularly detected hybrids correspond Christian Wehenkel, to those identified on a morphological basis. However, the morphology of P. herrerae [email protected] is not consistent with the molecularly identified hybrids from one population and is Academic editor only consistent with 3.3 of those from the other population. Tatiana Tatarinova Conclusions. This is the first report of hybrid vigour (heterosis) in Mexican pines. Additional Information and Information about hybridization and introgression is essential for developing effective Declarations can be found on page 19 future breeding programs, successful establishment of plantations and management of natural forest stands. Understanding how natural hybridization may influence the DOI 10.7717/peerj.8648 evolution and adaptation of pines to climate change is a cornerstone to sustainable Copyright forest management including adaptive silviculture. 2020 Wehenkel et al. Distributed under Creative Commons CC-BY 4.0 Subjects Agricultural Science, Genetics, Plant Science Keywords AFLP, Tree breeding, STRUCTURE, NewHybrids, Australes, Interspecific hybrids, OPEN ACCESS Tree species, Monitoring, Random Forest, Adaptive silviculture How to cite this article Wehenkel C, Mariscal-Lucero SRí, González-Elizondo MS, Aguirre-Galindo VíA, Fladung M, López-Sánchez CA. 2020. Tall Pinus luzmariae trees with genes from P. herrerae. PeerJ 8:e8648 http://doi.org/10.7717/peerj.8648 INTRODUCTION Hybridization represents an important evolutionary force that can introduce much more new genetic material than is created by mutation events (Anderson, 1949; Wright, 1964). It can also act as an additional, perhaps more abundant, source of adaptive genetic variation than mutation (Grant & Grant, 1994), by allowing gene flow and recombination (Abbott et al., 2013; Hipp, 2018). Furthermore, hybridization is one of the key sources of species formation and diversity, and many species may have originated by this route (Linder & Risenberg, 2004; Blanckaert & Bank, 2018), perhaps even as much as between 30% and 80% of all species (Wendel, McD & Rettig, 1991). On the other hand, increasing rates of hybridization may also lead to the extinction of unique populations or species because of unsuccessful reproductive efforts or introgression with a more common species (Rhymer & Simberloff, 1996; Blanckaert & Bank, 2018). In times of rapid ongoing climate change, hybridization may thus contribute to further extinctions, sometimes weakening reproductive isolation among species (Owens & Samuk, 2019) as well as supporting the development of novel segregating genotypes that will speed up adaptation to changes in climate (Chunco, 2014; Menon et al., 2019). Knowledge of hybridization has therefore deep practical reasons. Besides the adaptation issues, the presence of hybrid trees in seed stands ``contaminates'' the species' gene pool and thus may influence seed quality and afforestation success (Arnold & Hodges, 1995; Rieseberg & Carney, 1998). The process of hybridization incorporates alleles from one species into the gene pool of another (Harrison, 1993). Interactions between the environment and genetic structure can thus lead to segregation of a novel taxon from parental types. Depending on the degree of differentiation, hybrid offspring of two or more plants of different taxa are sometimes identified as species, subspecies or variants (Futuyma, 1998; Tovar-Sánchez & Oyama, 2004). Hybrids often display post-mating reproductive difficulties relative to their ancestors. These difficulties include hybrid weakness, sterility and fitness breakdown (Rieseberg & Carney, 1998). However, hybrids are not necessarily uniformly unfit. On the contrary, some genotypic classes may be equally fit or even fitter than the parental taxa (Arnold & Hodges, 1995; Mabaso, Ham & Nel, 2019). The first hybrid generation (F 1) tends to exceed the parental generation in vegetative vigour or robustness, in a condition also known as heterosis. However, early hybrid generations such as F 2 and F 3 are often less vigorous and fertile than their parents due to the break-up of adaptive gene arrangements (Rieseberg & Carney, 1998). Studies involving hybridization are often based on morphological traits. However, the phenotypic expression of characters of one taxon in another does not necessarily indicate hybridization. Similar characters may occur in species because of phenotypic plasticity, convergent evolution or simply because of a common ancestry, as Linder et al. (1998) observed in wild sunflower. Furthermore, morphological characters yield limited information when the parents and their hybrids are affected by environmental factors such as disease or drought stress, generating a wide range of phenotypic variability. This problem is increased by subsequent backcrossing of the hybrids to either parent species, resulting Wehenkel et al. (2020), PeerJ, DOI 10.7717/peerj.8648 2/28 in morphological characters that become more similar to those of the backcrossed parent species (Chen et al., 2004). Use of molecular markers to detect interspecific hybridization is more effective than verification by morphological, chemical or cytogenetic analysis, especially as access is available to an almost unlimited number of molecular markers (Rieseberg, Ellstrand & Arnold, 1993; Alexandrov & Karlov, 2018; Jasso-Martínez et al., 2018). Introgressive hybridization in many plant species has been identified by molecular data (Rieseberg, Ellstrand & Arnold, 1993; Arnold, 1997; Delgado et al., 2007; Kaplan & Fehrer, 2007; McVay, Hipp & Manos, 2017). These markers have been useful for diagnosing F 1 and derived hybrid generations, evaluating levels of gene flow among species and reconstructing phylogenetic relationships between hybridizing taxa and their close relatives (Rieseberg, Ellstrand & Arnold, 1993). Amplified Fragment Length Polymorphism (AFLP) markers have been successfully used to detect introgressive hybridization in plants (Guo et al., 2005; Shasany et al., 2005; Koerber, Anderson & Seekamp, 2013), specifically in pines (Xu, Tauer & Nelson, 2008; Stewart et al., 2010; Vasilyeva & Semerikov, 2014; Ávila Flores et al., 2016). AFLP markers include a more or less large number of polymorphic, di-allelic loci and can be developed relatively easily and at a relatively low cost, even for species about which no prior genetic information is available (Mueller & Wolfenbarger, 1999; Hardy et al., 2003; Paun & Schönswetter, 2012). Possible disadvantages of the AFLP technique such as compiling standardized patterns in a database for interlaboratory use and future reference can be avoided by using specific procedures as recommended by Savelkoul et al. (1999). However, AFLP as dominant marker does not allow identification of homologous alleles and thus scoring of homozygote and heterozygote states (Mueller & Wolfenbarger, 1999). Interspecific hybridization is very common in natural stands of the genus Pinus (Critchfield, 1967; Quijada et al., 1997; Conkle & Critchfield, 1988; López-Upton et al., 2001; Delgado et al., 2007; Ávila Flores et al., 2016; Stacy et al., 2017; Vasilyeva & Goroshkevich, 2018; Mo et al., 2019), because of very weak reproductive barriers between pine species (Little & Righter, 1965; Garrett, 1979; Dungey, 2001); this could be generalized across conifers with similar divergence history (Menon et al., 2018). Interspecific F 1 hybrids in this
Details
-
File Typepdf
-
Upload Time-
-
Content LanguagesEnglish
-
Upload UserAnonymous/Not logged-in
-
File Pages28 Page
-
File Size-