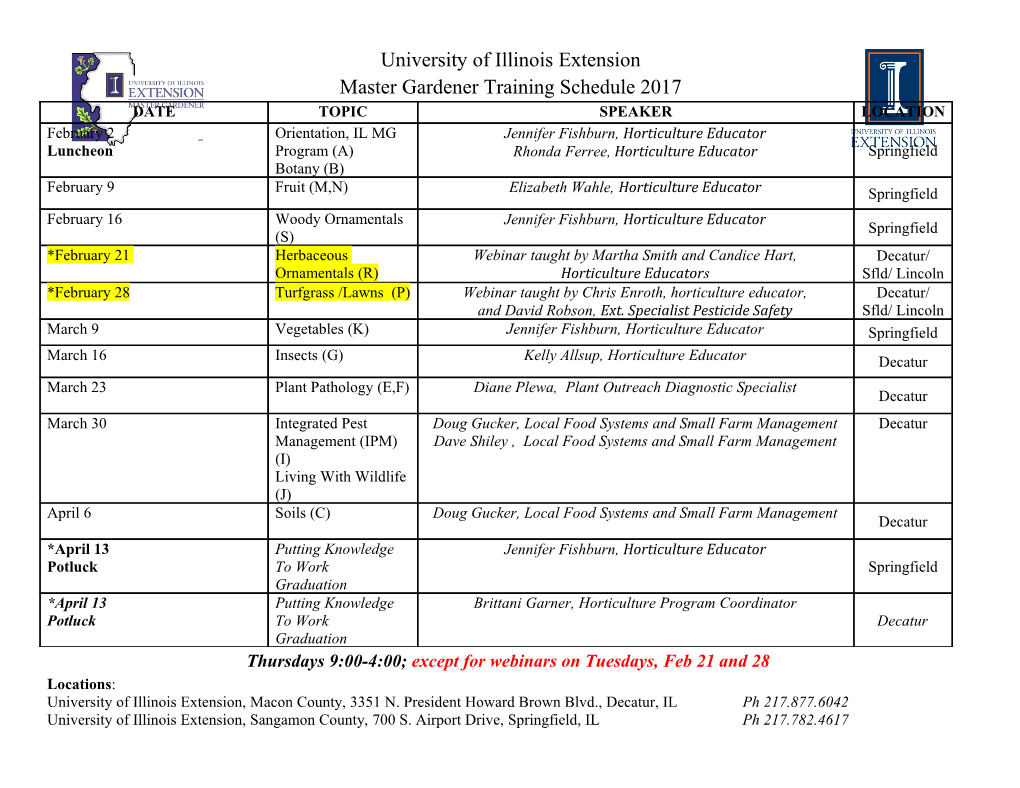
Final Report- Assessing Flood Risk in a Changing Climate in the Mohawk and Hudson River Basins Stephen Shaw SUNY College of Environmental Science and Forestry, Syracuse, NY The report is divided into five different sections: 1. Hudson River – Controls on Peak River Stage 2. Causative Processes of Flooding on Tributaries to the Main Stem of the Hudson and Mohawk 3. Controls on Precipitation Intensity in the U.S. and Hudson Valley 4. Ice Jams on the Mohawk River – A simple model to predict future ice jams 5. Fact Sheet for Public: “Mohawk River Flooding: Will the future differ from the past?” The first four sections reflect basic research that has gone into better understanding processes that dictate discharge and stage on the Hudson , Mohawk, and tributaries. Work from Sections 3 and 4 (precipitation intensity and ice jams) is ongoing. We plan to have publications on these two topics complete by the end of the spring. Work on Sections 1 and 2 is complete and provides some helpful conclusions that can provide insight into predicting potential future flooding. Section 5 includes a draft fact sheet that draws from the basic research of Sections 1 to 4. The fact sheet is not a summary of the basic research, but it instead borrows bits and pieces of the basic research to make points as necessary. We are happy to incorporate any suggested revisions into this document as we presume it will be the deliverable from this project most suitable for distributing to the general public or policy makers. Section 1 – Controls on Peak River Stage in the Hudson Unlike many rivers, the main channel of the Hudson River up to Albany has a very low channel slope and is sometimes likened to being similar to a fjord. The river is effectively an extension of the Atlantic Ocean. Thus, while in most rivers one thinks of stage (water height) as being dependent on inflow from the watershed, the stage in the mid-river of the Hudson can be dependent on variations in sea level due to tides, storm surge, and long-term changes. While recent storm surge due to Tropical Storm Sandy made people acutely aware of the extent storm surge could raise water levels in the Hudson River, one could ask the question: Do other factors, such as inflow from the watershed, matter at all in controlling Hudson River stage? If watershed inflows matter little, then predictions of how the frequency of high stage may change is dependent only on the frequency of high ocean stage and not on precipitation intensities, watershed wetness, or other factors that influence generation of runoff in the watershed. If ocean stage dominates mid-river stage, then predicting future frequency of high stages is somewhat simplified, requiring only accurate predictions of extremes in sea level. The only long-term measure of water level in the mid-Hudson is at Poughkeepsie. Therefore, to evaluate possible controls on mid-Hudson water stage we compared stage at Poughkeepsie to three possible controls: 1) ocean stage as indicated by the tidal gage at the Battery, Manhattan; 2) watershed inflows as represented by flow from the upper Hudson measured at Troy as well as from two gaged lower basin tributaries: the Walkill River and Wappinger Creek; and 3) sustained wind in the Hudson Valley given its potential to lead to wave run- up. We initially attempted to construct a multivariate regression model to relate these factors to mid-Hudson stage. We found that there were too many possible interactions and possibly missing variables (such as changes in water density) and that a statistical model could not sufficiently predict mid-Hudson stage. As an alternative, we used a qualitative approach to assess what factors were most important in leading to high mid-Hudson stage. This qualitative approach is shown in Tables 1 and 2. In Table 1 we indicate the 20 highest stage values at Poughkeepsie along with the concurrent values of the primary factors: Battery stage, watershed inflows, and wind speeds. Table 2 is similar but instead of using periods of highest mid-river stage we use the 20 dates with the lowest recorded stage value. Within each group of factors (e.g. stage at Battery, Troy Dam discharge, etc.) we established the range of the possible values and assigned percentiles. For instance we found that for daily discharge at the Troy dam, the 99th percentile value (the value that is met or exceeded 1 out of 100 days) was 60,000 cubic feet per second. Thus, we then color-coded the values of the primary factors to indicate the percentile of the value. Values at or exceeding the 99th percentile were colored red. Values between the 99th and 50th percentile were colored orange. The remainder were colored green. On Table 1, we see that high stage at Poughkeepsie is always accompanied by greater than 99th percentile value of at least one factor and at least 50th to 90th percentile values of additional factors. The three highest Poughkeepsie stage values occur when the Battery stage is above 99th percentile. In some cases where Battery stage values are not 99th percentile but Poughkeepsie stage is high, watershed discharge values are 99th percentile (4/2/05, 10/13/05, 4/16/07, 3/13/10). However, on days when watershed inflows are below 50th percentile (12/11/92, 5/12/08, 9/2/06), Poughkeepsie stage can still be very high as long as the Battery stage is also high. Thus, it appears that Battery stage dominates in controlling Poughkeepsie stage, although it does appear that very high watershed inflows have some moderate ability to enhance Poughkeepsie stage. Notably, wind appeared to also have some moderate influence. While wind speed was typically high, the wind direction also seemed critical. Namely, in Table 1, higher mid-river stage often occurred when winds were from the north or northeast. Winds could possibly be seasonally correlated to some other factor, but the importance of north or north east wind is consistent with the possibility that a steady north wind could push water down the Hudson and create increased wave heights in the mid to lower Hudson. When stage was at its lowest, the wind was consistently out of the west of northwest. The fact that multiple processes interact to control river stage has also recently been detailed by Orton et al. 2012 (Detailed modeling of recent severe storm tides in estuaries of the New York City region, Geophysical Research Letters – Oceans, 117, C9) using a dynamic model of the estuary. Orton et al. were able to run the model with certain processes removed to assess how the simulations of river stage were influenced. For Tropical Storm Irene, they found that removing watershed inputs under-predicted river stage at Poughkeepsie by approximately 18%, consistent with our assessment that watershed inputs have a moderate influence on mid- Hudson stage. They noted that this influence increased as one moved upriver and indicated (Orton et al. 2012 Figure 15) that near Albany watershed inflows could dictate up to 50% of stage height. Table 1. Stage at Battery, watershed discharge, and winds at the same time as the twenty highest recorded mid- river stage values. Red shading indicates 99th percentile value. Orange shading indicates 99th to 50th percentile value. Green indicates a value below the 50th percentile. Airport: Avg. Wind Avg. Wind Direction Stage (NGVD 1929 ft) Discharge (cfs) Wind Speed (mph) (deg. from N) Cardinal Hudson @ Ocean @ Hudson Wallkill Wappinger Dutchess Stewart Dutchess Stewart Date of Peak Dir. Poughkeepsie Battery @ Troy River Creek Co. Int'l Co. Int'l 10/29/12 4.36 6.1965 - 1630 294 17.58 23.71 24.18 46 N/NE 12/11/92 4.33 4.6565 16000 2080 262 14.41 23.42 70 56.36 E/NE 4.32, 3.49, 8/28-30/11 3.0415 158000 21100 7320 14.65 21.32 n/a n/a n/a 3.15 4/2-3/05 3.23, 4.22 2.709 108000 17500 2210 11.77 14.94 n/a n/a n/a 4.2, 3.84, 4/16-19/07 2.389 104000 21300 7640 12.53 14.76 10.14 30.42 N 3.52, 3.36 10/28/06 3.65 2.4315 56500 6770 551 11.32 17.02 n/a n/a n/a 10/25/05 3.63 3.7665 80500 4910 2090 12.44 16.02 359.78 33.64 N 12/21/12 3.53 2.534 - 4830 796 13.13 18.25 n/a n/a n/a 10/19-20/96 3.5, 3.07 3.619 32000 9680 1950 12.27 21.30 75.24 76.25 E 3/13-14/10 3.11, 3.43 3.564 44000 12400 1270 12.93 19.48 39.6 75.71 NE/E 10/13/05 3.41 3.949 22300 15500 2180 7.14 13.00 355.5 48.75 N/NE 9/8-9/11 3.35, 3.33 2.739 107000 22200 4940 4.70 9.06 n/a 72.14 E 3/11/11 3.35 2.2915 76300 15300 3950 7.74 14.94 n/a n/a n/a 1/25/10 3.23 2.1915 56900 5530 1290 10.33 16.80 152.28 157.3 SE 5/12/08 3.22 3.5315 15500 1240 218 10.60 16.49 43 67.2 NE 9/2/06 3.21 3.329 9980 2020 146 10.74 16.04 n/a 76.82 E 1/18/06 3.17 1.179 87400 7890 2320 13.35 19.93 n/a n/a n/a 11/28/93 3.08 2.024 29800 4070 857 14.41 16.62 151 150.5 SE 12/14/92 3.05 2.919 13800 2000 230 n/a 8.30 n/a 49.17 NE 11/11/10 3.04 2.724 23800 725 204 8.54 9.06 17.39 58.10 N/NE 12/11/03 3.04 1.8715 59300 6730 1960 - - - - - 5/12/98 3.02 2.659 42400 9070 2030 12.73 17.16 46.875 61.74 NE Table 2.
Details
-
File Typepdf
-
Upload Time-
-
Content LanguagesEnglish
-
Upload UserAnonymous/Not logged-in
-
File Pages20 Page
-
File Size-