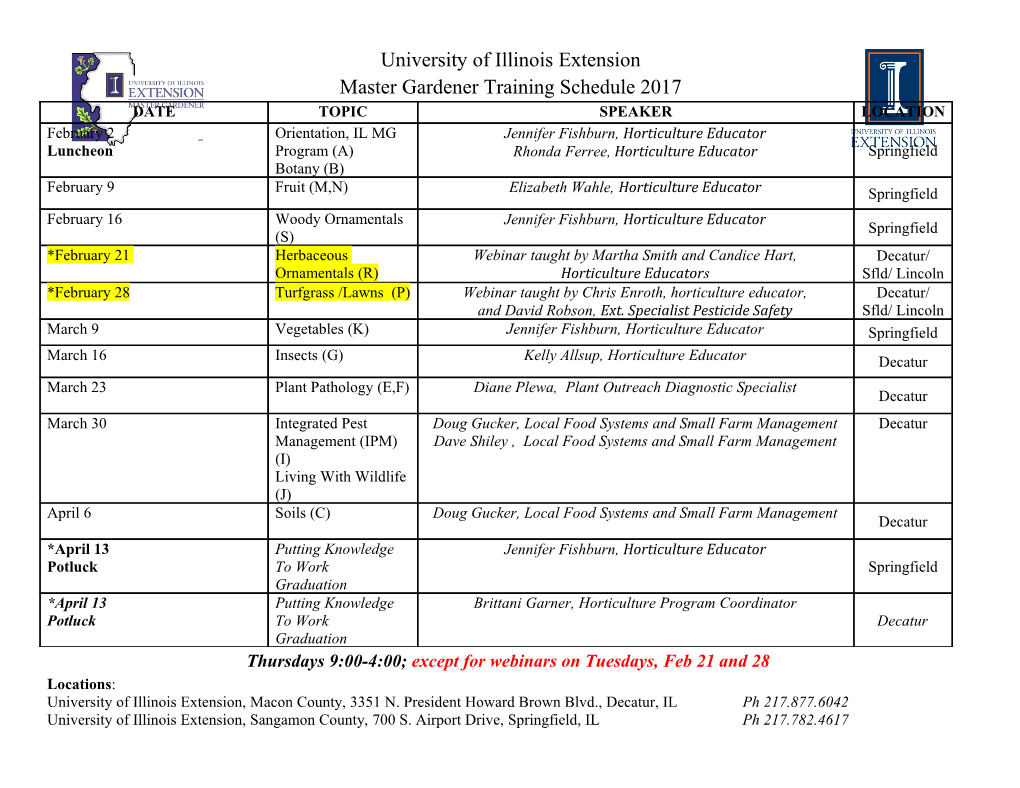
AN ABSTRACT OF THE THESIS OF Shawn P. Bennett for the degree of Master of Science in Atmospheric Sciences presented on September 10. 1987 Redacted for privacy Abstract approved: Steven A. Rutledge On May 28, 1985 a nocturnal esosca1e convective ystem (MCS) moved into the observational network of the O.K. PRE-STORM (Oklahoma-Kansas .reliminary egionalxperiment for STORM-Central) Project. Satellite imagery showed an extensive circular cloud shield. Sensing of meteorological parameters by the NCAR (National Center for Atmospheric Research) Portable Automated Mesonet (PAM II), as well as observations by both conventional and Doppler radar document this MCS as a fast moving squall line. This MCS developed along the Nebraska-Wyoming border in the warm moist air associated with a quasi-stationary frontal boundary parallel to the Front Range of the Rocky Mountains. In addition to the lifting caused by the frontal boundary, the upsiope topography of the Central Great Plains as it rises to meet the Front Range of the Rocky Mountains caused additional instability by orographically lifting the convectively unstable air mass. Initial development of the MCS may have been forced by an indirect circulationin the left-front exit zone of a 25 m jet streak over the region at 300 mb (Ucellini and Johnson, 1979) . At the surface a lee-side trough was found along the Front Range, while aloft a broad long wave ridge was positioned over theCentral U.S. Changes created in the near surface boundary layer environment during the passage of the MCS are documented by the PAN II mesonetwork. A mesohigh associated with the convective line and a wake lowor "wake depression" (Fujita, 1955; Johnson and Hamilton, 1987) were observed. Analysis of single and dual-Doppler radar data reveal the kinematic structure and radar reflectivity of the MCS from near the surface boundary layer to the upper troposphere. Several features are identified, including a cyclonic mid-level circulation - 7 km deep (Fritsch and Chappell, 1980), a plunging rear inflow jet with relative flow speeds of -10-15 ms-, an echo-free notch in the rear of the stratiform region, and a bow echo in the convective line (Smull and Houze, 1985; 1987a,b) The 12-hour-plus duration of the MCS may be attributed to a combination of positive feedback mechanisms which reinforced the squall line with positive vertical momentum. Among these positive feedback mechanisms was the configuration of the surface mesoscale pressure systems which may have increased the low-level horizontal convergence and as a result supplied additional mass and moisture to the convective line. This then may build the convective cells within the line and lead to an intensification of the mesoscale pressure systems and induce more convergence, etc (Fritsch and Chappell, 1980) A Case Study of the May 28, 1985 MesoscaleConvective System Observed During the O.K. PRE-STORM Project by Shawri P. Bennett A THESIS submitted to Oregon State University in partial fulfillment of the requirements for the degree of Master of Science Completed September 10, 1987 Commencement June, 1988 APPROVED: Redacted for Privacy Professor of Atmospheri Sciences in charge of major Redacted for Privacy Chairman of Depa(entof AtmosphericSciences Redacted for Privacy Dean of Graduate7choo1 Date thesis is presented September 10, 1987 Presented by Shawn P. Bennett ACKNOWLEDGEMENTS I would like to thank my MajorProfessor, Dr. Steven A. Rutledge, for his valuablerecommendations and guidance during the preparation of this thesis. I also appreciate the comments by members of my thesiscommittee Drs. Steven K. Esbensen, Glenn A. Klein, and Peter R. Fontana I would like to thank Dean A.Vickers for his helpfulness and expertise in computerprogramming, data aquisition, and data analysis techniques. Special thanks are in order for Steven M. Ginn for his helpin computer programming. This research was supported by the NationalScience Foundation under grants ATM-8317466 and ATM-8521403. TABLE OF CONTENTS 1. INTRODUCTION 1 1.1 General Background and Earlier Studies of Convective Storms 1 1.2 Recent Studies of Mesoscale Convective Systems 6 1.3 Recent Studies Using Doppler Radar Observations 10 1.4 Research Objectives 13 2. PRE-STORM PROGRAM DESIGN AND OPERATIONS PLAN 15 2.1 Program Background and Goals 15 2.2 Observational Program Design 16 3. SYNOPTIC AND SATELLITE OVERVIEW 22 3.1 Surface Synoptic Situation 22 3.1.1 0600 GMT 28 May 1985 meteorological conditions 22 3.1.2 1200 GMT 28 May 1985 meteorological conditions 23 3.1.3 1800 GMT 28 May 1985 meteorological conditions 25 3.2 Upper-Air Long and Shortwave Pattern 27 3.2.1 0000 GMT 28 May 1985 upper-air pattern 27 3.2.2 1200 GMT 28 May 1985 upper-air pattern 30 3.3 Classification and Definition of MCSs 34 3.4 Satellite Imagery of the 28 May 1985 MCS 37 4. MESOSCALE ANALYSIS 47 4.1 Discussion of the WSR-57 Radar Observations 47 4.2 The PAN II Surface Mesonetwork 54 4.2.1 GEMPAK and PAN II data analysis methods 57 4.2.2 Analysis of PAN II data 58 4.2.3 Surface mesoscale pressure systems 74 4.3 Analysis of Single Station Time Series 80 5. SINGLE DOPPLER ANALYSIS 86 5.1 Introduction 86 5.2 Discussion of Vertical Cross-Section Analysis 86 5.3 Discussion of Velocity-Azimuth-Display (VAD) Analysis 106 5.4 Discussion of Vertically-Pointing (VP) Analysis 115 6. DUAL-DOPPLER ANALYSIS OF THE TRAILING STRATIFORN REGION AND ASSOCIATED MESOSCALE CIRCULATION 121 6.1 Discussion of Reflectivity and Kinematic Fields 122 6.2 Summary of Evidence Regarding a Mesoscale Circulation 130 7. CONCLUSIONS 132 7.1 Conclusionsfrom the Observational Analysis 132 7.2 Suggestionsfor Further Research 135 REFERENCES 137 LIST OF FIGURES 1.1. Tracks of MCSs during May, June,July of 1979 through 1983. Dotted lines indicate position of early storm development. Circled numbers correspond to the position of themaximum extent of the -32CC cloud shield from infrared satellitedata. The 'X'1 represents the location where theconvective system was terminated as a MCS. (Adapted from Cunning, 1986). 2 1.2 A schematic section through a squallline thunderstorm illustrating the horizontaland vertical structures of the squall line. The wake depression, the internal airflow (thin arrows), and environmental airflow (broad arrows) are depicted. (Adapted from Fujita, 1955). 5 1.3. Time cross section through the 29 May 1947 squall line during its passage over the Thunderstorm Project Network near Wilmington, Ohio. Thin solid lines are wet-bulb potential temperature (deg K); heavy lines, boundaries of stable layersassociated with squall front on right, and polar front onthe left. Arrows indicate schematic stormrelative average circulation. Stippled region is cloud. (Adapted from Newton and Newton, 1959). 7 1.4. Conceptual model of the 22 May 1976 squallline system at maturity. Outermost scalloped line is extent of cloud. Outermost solid line is extent of radar echo useable for Doppler velocity measurements. Heavy solid lines are more intense reflectivity features. Mesohigh and wakelow are located at "H" and "L". Storm relative air motions are shown by solid arrows. Broad arrow from asterisk indicates hypothesized ice particle trajectory. (Adapted from Smull and Houze, 1985). 12 1.5. Revised conceptual model based upon new research findings. Note airflow changes, especially those associated with the convective tower. (Adapted from Smull and Houze, 1987b). 12 2.1. Overview of the surface observational mesonetwork in Kansas and Oklahoma during PRE-STORM. 17 3.1. 0600 GMT 28 May 1985 Synoptic analysis 24 3.2 1200 GMT 28 May 1985 Synoptic analysis 24 3.3 1800 GMT 28 May 1985 Synoptic analysis 26 3.4 0000 GMT 28 May 1985 850 mb level analysis 26 3.5 0000 GMT 28 May 1985 700 mb level analysis 29 3.6 0000 GMT 28 May 1985 500 mb level analysis 29 3.7 0000 GMT 28 May 1985 300 mb level analysis 31 3.8 1200 GMT 28 May 1985 mb 850 level analysis 31 3.9 1200 GMT 28 May 1985 700 mb level analysis 33 3.10 1200 GMT 28 May 1985 500 mb level analysis 33 3.11 1200 GMT 28 May 1985 300 mb level analysis 35 3.12Satellite infrared image showing the enhancement curve temperature ranges that correspond tothe various shades of black, gray, and white. Colder cloud tops infer stronger more intenseconvection. (Adapted from Maddox, 1980). 36 3.13 0000 GMT 28 May 1985 infrared satelliteimage 36 3.140300 GMT 28 May 1985 infrared satelliteimage 38 3.15 0600 GMT 28 May 1985 infrared satelliteimage 38 3.16 0900 GMT 28 May 1985 infrared satellite image 40 3.17 1101 GMT 28 May 1985 infrared satellite image 40 3.18 1331 GMT 28 May 1985 visible satellite image 42 3.19 1501 GMT 28 May 1985 infrared satellite image 42 3.20 1800 GMT 28 May 1985 infrared satellite image 44 3.21 1830 GMT 28 May 1985 infrared satellite image 44 3.222300 GMT 28 May 1985 infrared satellite image 45 4.1. Observed reflectivity at 1008 GMT 28 May 1985from Wichita NWS WSR-57 radar. The elevation angle of this PPI and all that follows is10. Light to darker gray shading every 10 dBZ beginningwith 20 dBZ. Contour interval 10 dBZ beginning with 10 dBZ. 48 4.2 As in fig. 4.1 except for 1127 GMT. 50 4.3 As in fig. 4.1 except for 1356 GMT. 52 4.4 As in fig. 4.1 except for 1457 GMT. 53 4.5 The Oklahoma-Kansas PRE-STORM PAN II mesonetwork. (Adapted from Johnson and Toth, 1986) . 55 4.6 NCAR Portable Automated Mesonetwork Station. (Adapted from Johnson and Toth, 1986). 56 4.7 Mesoanalysis of PAN II data at 1010 GMT 28 May 1985.
Details
-
File Typepdf
-
Upload Time-
-
Content LanguagesEnglish
-
Upload UserAnonymous/Not logged-in
-
File Pages161 Page
-
File Size-