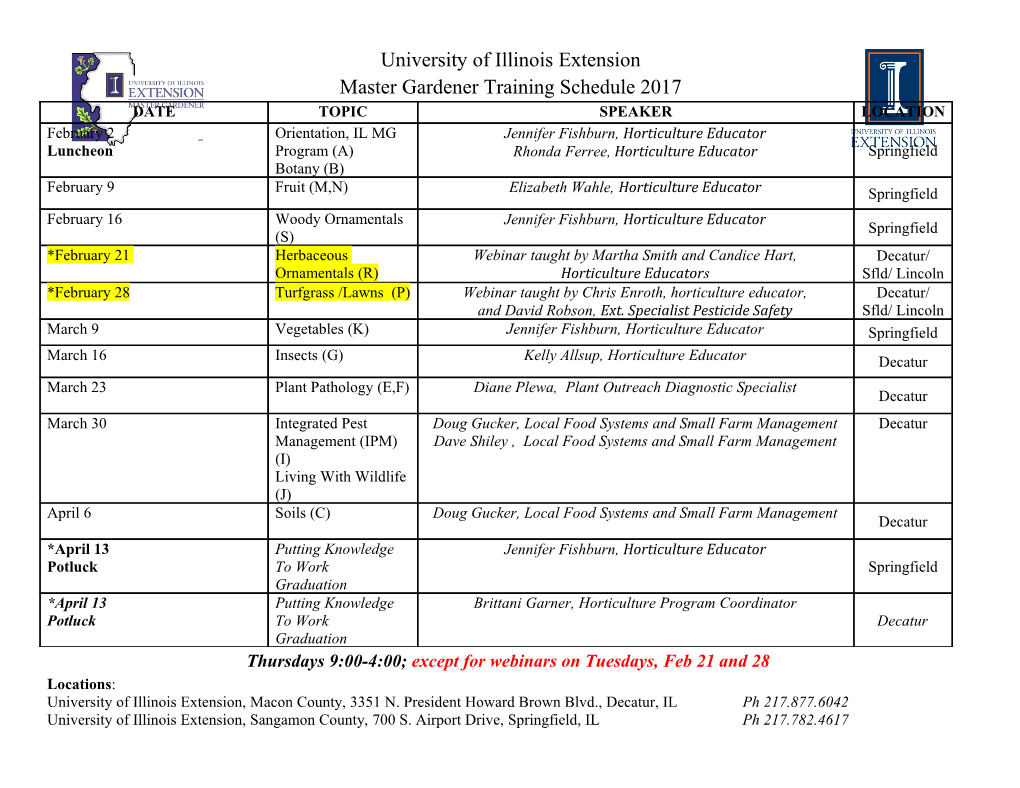
Physics 106 Lecture 12 Oscillations – II SJ 7th Ed.: Chap 15.4, Read only 15.6 & 15.7 • Recap: SHM using phasors (uniform circular motion) • Phys ica l pendu lum example • Damped harmonic oscillations • Forced oscillations and resonance. • Resonance examples and discussion – music – structural and mechanical engineering – waves • Sample problems • Oscillations summary chart Phasors: The x-component of uniform circular motion looks just like simple harmonic motion (oscillation) A vector rotating at constant angular speed y G v(t) v = ω r G G a(t) G r(t) 2 v(t) G a = ω r G r(t) a(t) ωt + ϕ x 1 Phasors: The x-component of uniform circular motion looks just like simple harmonic motion (oscillation) x(t) = xm cos(ωt + ϕ) v(t) is perpendicular to x(t) vx (t) = −ωxm sin(ωt + ϕ) a(t) is along -x(t) vmax = −ωxm G a(t) is the centripetal r(t) accelileration 2 ax (t) = −ω xm cos(ωt + ϕ) 2 ax,max = −ω xm 2 Oscillator d x(t) 2 Equation: ≡ −ω x(t) dt2 The physics is in determining ω and the amplitude Velocity of an oscillator 12.1 A spring oscillator with mass m = 10.0 kg is in simple harmonic motion with x(t) = (3π)cos(10πt), where x(t) is the displacement in meters and t is in seconds. How much time does it take for the block to move from one point of zero acceleration to the next such point? A) 0.15 s B) 0.20 s C) 0.25 s D) 0.10 s E) 0.30 s xt( ) = A cos (ω t+ φ ) dx vA==−ωωφsin( t + ) dt dx2 aA==−ω 2 cos(ωφ t + ) dt 2 2 Repeating: Simple Pendulum…. • Mass m swinging at the end of a massless string • Angular displacement θ(t) oscillates: − θm ≤ θ ≤ +θm • Gravity provides restoring torque: τ = − m g L sin(θ) 2 • Apply angular d θ 2 τ = Ια = Ι (force equation) Ι ≡ mL Second Law: dt2 • AlApply sma ll θ θ3 θ5 sin(θ) = θ − + − ..... ≈ θ (radians) approximation: 3! 5! mgL no ∴ α(t) ≈ − θ(t) torque mL2 d2θ(t) g • Another oscillator equation: torque = − θ(t) acceleration and displacement 2 dt L have same time dependence phase constant • Solution is displacement θ(t) = θ cos (ωt + φ) (radians) oscillations: at t m amplitude natural frequency natural g ω ≡ no mass dependence or amplitude dependence! frequency L What if pendulum is NOT a simple point mass as above? • Moment of inertia Ι is not mL2……………………… • Call it a “physical pendulum The Physical Pendulum – Not a point mass so I is important! • Mass m acts as if it is concentrated at center of gravity C • Pivoted at “O”, a distance h from the CG • Displacement θ(t) oscillates if h ≠ 0 Amplitude: ± θm • Rotational Inertia Ι about “O” depends on shape: 2 Ι ≠ mL [not simple pendulum] • Restoring torque using small angle approximation: τ = − m g h sin(θ) ≈ − mghθ(t) torque no • Apply angular Second Law: d 2 torque τ = Ι α ( t ) = Ι θ ( t ) dt 2 • Another d2θ(t) mgh oscillator 2 = − θ(t) equation: dt Ι The equation and solution are the same as for the simple pendulum, but with a different frequency! mgh 2π Ι Solution θ(t) = θ max cos( ω t + φ) where ω ≡ T = = 2π Ι ω mgh • h Æ 0 means suspended at CG, so ω Æ 0 (no oscillation) • Ι Æ mL2 recovers simple pendulum • Does ω depends on mass? 3 Example: a uniform rod used as a physical pendulum: suspended at one end, length L • For uniform rod, h = L/2, oscillations are “small” h L • Rotational Inertia about rotation axis at end of rod: θ(t) 2 1 2 2 1 2 CG Ι = Ι + mh = mL + mh = mL end cm 12 3 •Second Law and torque due to weight of rod at CG: mg Ια = τnet = - mgh si(in(θ) ≈ - mgh θ(t) oscillator d2θ(t) mgh solution as = − θ(t) equation: dt2 Ι before angular 2 mgh mg L/2 3 g 2 g for simple ω ≡ = = versus ω = frequency: Ι 1/3 m L2 2 L L pendulum • No dependence on rod’s mass or on amplitude of oscillation Example: Find the period for a uniform meter stick • L = 1 m., mass m won’t matter, Ι = 1/3 mL2 and h = L/2 as above, small oscillations 2π Ι 2L 2 1.0 T ≡ = 2π = 2π = 2π = 1.64 sec for ANY meter stick ω mgh 3g 3 9.8 Simple pendulum with 2 3 g g 2 ω = ≡ L0 = L = 0.66 m same period has length L0: 2 L L0 3 Equivalent simple and physical pendula 12.2 A certain physical pendulum has an angular frequency of 2.0 rad/sec. What would be the length of a simple pendulum with the same period?* A) 193 m B) 2.45 m C) 4.90 m D) 1.57 m E) h = ω2I/gh *This is called the “center of oscillation” mgh 2 g ω2 ≡ ω = Ι L0 4 Torsion Pendulum SHM involving angular coordinate • A restoring torque acts when τ = −κθ (κ positive) disk is twisted from rest: • Device oscillates − θ ≤ θ ≤ +θ with amplitude: m m • Apply Second Law angular): d2θ τ = Iα = I (force equation) 2 I dt d2θ(t) κ • Oscillator equation form: = − θ(t) acceleration and displacement dt2 I have same time dependence • Solution oscillates, as did linear oscillator: phase constant angular displacement (radians) at time t θ(t) = θm cos (ωt + φ) amplitude natural frequency natural κ frequency ω ≡ no amplitude Note: θ(t) = displacement Ι dependence! ωt+φ = “phase” 2π Ι does depend on φ = “phase constant” period T = = 2π ω κ mass distribution I Damped Oscillations • Non-conservative forces may be present – Friction is a common nonconservative force neglect – No longer an ideal system (such as those dealt with gravity so far) • The mechanical energy of the system diminishes in time, motion is said to be damped • The motion of the system can be decaying oscillations if the damping is “weak”. • If damping is “strong”, motion may die away without oscillating. • Still no driving force, once system has been started 5 Add Damping: Emech not constant, oscillations not simple • Spring oscillator as before, but with dissipative force Fdamp velocity dependent not constant neglect viscous drag friction force gravity Fdamp = −bv b depends on fluid’s density and viscosity- - large for grease, small for air [b] = kg/s Acceleration proportional to speed • Previous force equation gets a new , damping force term d2x(t) dx(t) Fnet = m = − kx(t) − b dt2 dt new term d2x(t) b dx(t) k + = − x(t) dt2 m dt m bt − 2 Solution: 2m k b x(t) = xme cos(ω't + φ) ω'≡ − modified m 2 oscillations exponentially altered ω’ can be real 4m decaying envelope frequency or imaginary • Recover undamped solution for b Æ 0 • Critical damping Æ ω’ = 0, x(t) decays exponentially, no oscillation • For very strong damping (overdamping) ω’ is imaginary, x(t) decays faster than exponential b2 k cosine oscillations • Weak damping means: << ≡ ω2 for which ω'≈ ω 2 0 0 within an exponential 4m m envelope Damped physical systems can be of three types bt − 2 Solution: 2m k b x(t) = xme cos(ω't + φ) ω'≡ − damped m 2 exponentially altered ω’ can be real 4m oscillations decaying envelope frequency or imaginary 2 Underdamped: b k 2 << ≡ ω0 for which ω'≈ ω0 4m2 m The restoring force is large compared to the damping force. The system oscillates with decaying amplitude 2 Critically damped: b k 2 ≈ ≡ ω0 for which ω'≈ 0 4m2 m The restoring force and damping force are comparable in effect. The system can not oscillate; the amplitude dies away exponentially 2 Overdamped: b k 2 > ≡ ω0 for which ω' is imaginary 4m2 m The damping force is much stronger than the restoring force. The amplitude dies away as a modified exponential Note: Cos( ix ) = Cosh( x ) 6 Types of Damping, cont a) an underdamped oscillator b) a critically damped oscillator c) an overdamped oscillator For critically damped and overdamped oscillators there is no periodic motion and the angular frequency ω has a different meaning Weakly damped (“underdamped”) oscillator - velocity Amplitude : Xm = A 2 b k 2 bt << ≡ ω0 - 4m2 m 2m xm(t) ≈ xme small fractional slow decay change in amplitude of amplitude envelope during one complete cycle ≈ cos(ω0t + φ) Velocity with weak damping: find derivative bt − d 2m maximum velocity v(t) = x(t) ≈ vme sin(ω't + φ) dt vm = − ω0xm altered exponentially frequency ~ ω decaying envelope 0 7 Mechanical energy decays exponentially in an “underdamped” oscillator E = K(t) + U(t) = 1 mv2(t) + 1 kx2(t) mech 2 2 Substitute previous solutions: bt bt − − 2m 2m x(t) = xme cos(ω't + φ) v(t) ≈ −ω0xme sin(ω't + φ) E = 1 mω2x2 e−bt / msin2(ω't + φ) mech 2 0 m + 1 kx2 e−bt / mcos2(ω't + φ) 2 m As always: cos2(x) + sin2(x) = 1 2 k Also: ω ≡ 0 m ∴ E (t) = 1 kx2 e−bt / m mech 2 m exponential decay at twice Initial mechanical energy the rate of amplitude decay Forced (Driven) Oscillations and Resonance An external driving force starts oscillations in a stationary system The amplitude remains constant (or grows) if the energy input per cycle exactly equals (or exceeds) the energy loss from damping Eventually, Edriving = Elost and a steady-state condition is reached Oscillations then continue with constant amplitude Oscillations are at the driving frequency ωD ω0 = natural frequency (resonant frequency) ωD = driving frequency of external force External driving force function: FD(t) = F0 cos(ωDt + φ') FD(t) Force acts on damped oscillator equation: d2x(t) dx(t) FD(t) = m + b + k x(t) dt2 dt The system always oscillates at the driving frequency ωD in steady-state The amplitude A depends on how x(t) = Acos(ωDt + φ) close ωD is to resonance at ω0 8 Forced Oscillations and Resonance F /m Amplitude of the driven oscillations: A = 0 bω (ω2 − ω2 )2 + ( D )2 The largest amplitude oscillations occur at D 0 m or near RESONANCE (ωD ~ ω0) resonance At resonance, the applied force is in phase with the velocity and the power Fov transferred to the oscillator is a maximum.
Details
-
File Typepdf
-
Upload Time-
-
Content LanguagesEnglish
-
Upload UserAnonymous/Not logged-in
-
File Pages10 Page
-
File Size-