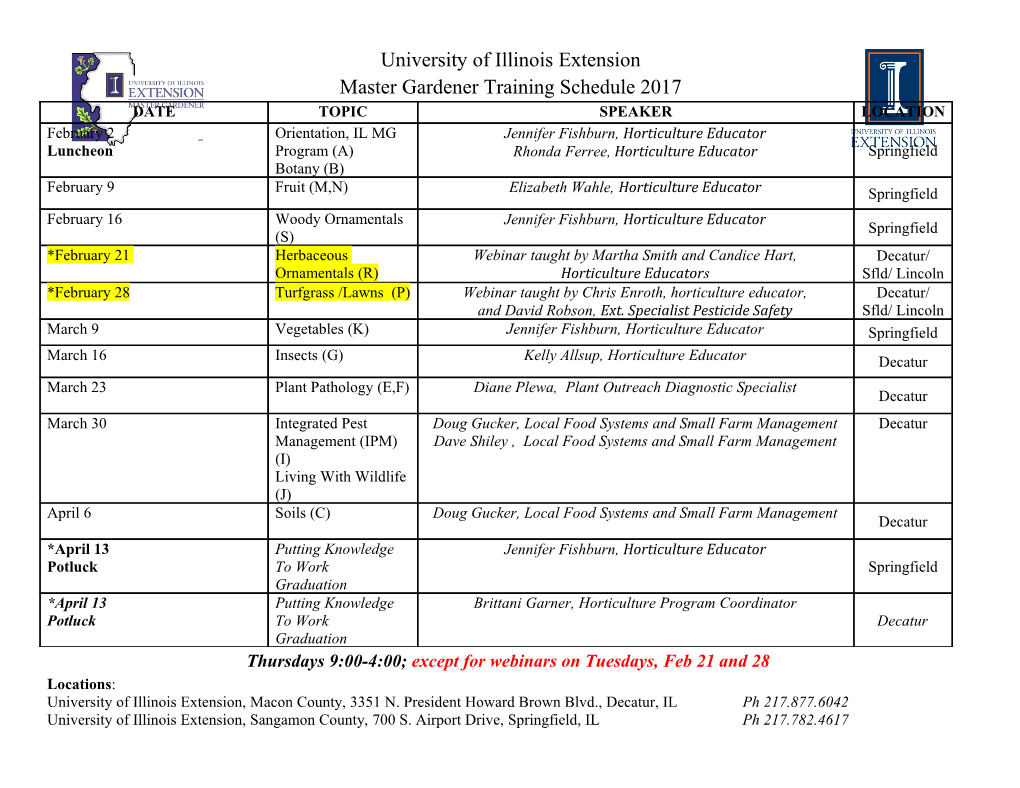
PROTOCOL Quantitative monitoring of mouse lung tumors by magnetic resonance imaging Alexander Sasha Krupnick1,5, Vanessa K Tidwell2,5, John A Engelbach3, Vamsi V Alli1, Arye Nehorai2, Ming You4, Haris G Vikis4, Andrew E Gelman1, Daniel Kreisel1 & Joel R Garbow3 1Department of Surgery, Washington University in St. Louis, St. Louis, Missouri, USA. 2Department of Electrical and Systems Engineering, Washington University in St. Louis, St. Louis, Missouri, USA. 3Department of Radiology, Washington University in St. Louis, St. Louis, Missouri, USA. 4Medical College of Wisconsin Cancer Center, Milwaukee, Wisconsin, USA. 5These authors contributed equally to this work. Correspondence should be addressed to J.R.G. ([email protected]). Published online 5 January 2012; doi:10.1038/nprot.2011.424 Primary lung cancer remains the leading cause of cancer-related death in the Western world, and the lung is a common site for recurrence of extrathoracic malignancies. Small-animal (rodent) models of cancer can have a very valuable role in the development of improved therapeutic strategies. However, detection of mouse pulmonary tumors and their subsequent response to therapy in situ is challenging. We have recently described MRI as a reliable, reproducible and nondestructive modality for the detection and serial monitoring of pulmonary tumors. By combining respiratory-gated data acquisition methods with manual and automated segmentation algorithms described by our laboratory, pulmonary tumor burden can be quantitatively measured in approximately 1 h (data acquisition plus analysis) per mouse. Quantitative, analytical methods are described for measuring tumor burden in both primary (discrete tumors) and metastatic (diffuse tumors) disease. Thus, small-animal MRI represents a novel and unique research tool for preclinical investigation of therapeutic strategies for treatment of pulmonary malignancies, and it may be valuable in evaluating new compounds targeting lung cancer in vivo. INTRODUCTION Pulmonary tumors remain a major source of morbidity and Computerized tomography mortality in our society1–3. Mouse models have a crucial role in CT is the imaging modality of choice for screening, diagnosis and advancing our understanding of malignancies and testing thera- monitoring of lung cancer in humans because of its high resolu- peutic options before initiating clinical trials. For example, recent tion, speed of data acquisition and ability to detect subcentimeter clinical success in the blockade of cytotoxic T lymphocyte antigen-4 nodules24,25. On the basis of this success in humans, multiple for treatment of malignant melanoma4,5 can be linked directly to groups have adapted micro-CT to mouse models of lung cancer related preclinical small-animal studies demonstrating the success and have used this technology for both detection and serial moni- of such a therapy6. Most of these preclinical experiments, however, toring of the disease11,26. However, micro-CT can deliver radiation rely on the subcutaneous injection of tumor cell lines, and response in doses ranging from 0.22 to 0.76 Gy per scan20,27. Although such to therapy is evaluated by the direct measurement of tumor dia­ radiation doses are several log-fold lower than the therapeutic 6,7 28,29 © All rights reserved. 2012 Inc. Nature America, meter . Although this approach may be valid for malignancies of doses typically used to treat lung cancer , the possibility that the skin or soft tissue, it does not translate directly to the physio­ radiation delivered by micro-CT can affect tumor growth, espe- logy of tumors that develop and grow in the lung because of consi­ cially with serial imaging, must be considered. In addition, the derable differences in immunosurveillance between the lung and cumulative effects of serial CT scans on the immune system need subcutaneous sites8,9. to be taken into account when designing long-term drug studies. Traditional models for the study of orthotopic mouse lung cancer Doses of whole-body irradiation as low as 2.7–4.2 Gy (5–6 (i.e., tumors occurring within the lung) have relied on either the micro-CT scans) can result in peripheral lymphopenia, lead- administration of carcinogen10 or intratracheal or intravenous (i.v.) ing to homeostatic proliferation of the remaining native T lym- injection of lung cancer cell lines, which result in intrapulmonary phocytes as they expand to repopulate the immune system30–32. tumor growth11,12. The recent development of genetically engi- As T cell homeostatic proliferation can lead to a tumor-immune neered mouse models of lung cancer (e.g., activation of oncogenic response33, even low doses of radiation may confound therapeutic K-ras, loss-of-function of p53) has substantially furthered the studies. Although both PET scanning and BLI can provide infor- range of experimental tools available for modeling lung cancer and mation regarding tumor burden, either by uptake of a radioactive offered a reproducible and reliable system for studying biological tracer (PET) or by detection of tumors engineered to express and therapeutic strategies for treating lung cancer13–20. luciferase (bioluminescence), the anatomic resolution of both Many investigators have relied on destructive modalities, such modalities is low (Table 1)23,34 (e.g., typical spatial resolution of as killing animals and analyzing tumor burden by counting tumor small-animal PET is 1–2 mm (ref. 35)). Despite these limita- nodules on lung blocks or histological slides14,21, for monitoring tions, all three of these imaging modalities have had an important intracavitary malignancies, such as lung cancer, and their response role in preclinical small-animal studies of tumor therapy20,23,26. to therapy. The use of nondestructive imaging modalities such as However, an imaging modality with high anatomic resolution computerized tomography (CT) scanning11,20, bioluminescent that avoids ionizing radiation, and that requires neither introduc- imaging (BLI) of tumor cells expressing a marker gene such as tion of foreign genes into the tumor nor extraneous manipula- luciferase22, or metabolic imaging such as positron emission tom- tion of a tumor cell line, may be advantageous for small-animal ography (PET) scanning, has also been reported23 (Table 1). studies of lung cancer. 128 | VOL.7 NO.1 | 2012 | NATURE PROTOCOLS PROTOCOL TAbLE 1 | Comparison of modalities for noninvasive in vivo imaging of pulmonary tumors. Modality Strengths Weaknesses References MRI Subject not exposed to ionizing radiation Requires respiratory gating 23,42,43,45, Excellent endogenous image contrast between Requires access to a small-animal MRI scanner 46,48,51,52 tumor and healthy lung tissue or purpose-built small-animal RF coil for High spatial resolution, with ability to quantify clinical scanner changes in spatial and temporal tissue morphology Not high throughput (~40 min per subject) Visualization of vasculature using dynamic contrast-enhanced imaging Micro-CT High spatial resolution Subject exposed to ionizing radiation 23,27,48,53 No need for respiratory gating with free-breathing Requires access to a small-animal CT scanner technology, but preferred for high resolution Visualization of vasculature requires a large dose of High throughput (~10 min per subject) contrast agent Excellent endogenous image contrast between tumor and healthy lung tissue Micro-PET Metabolic evaluation of primary tumor and Subject exposed to ionizing radiation 23,48,54,55 systemic metastasis Low spatial resolution, lack of anatomic information High signal detection sensitivity Requires synthesis and administration of Quantitative measurement of uptake kinetics radiolabeled tracers Requires access to a small-animal PET scanner Optical Subject not exposed to ionizing radiation Low spatial resolution at depths > 500 µm, 14,23,46, Relatively inexpensive, compact benchtop lack of anatomic information 56–60 or portable instrumentation BLI requires expression of biomarker High-throughput determination of tumor (luciferase) by tumor cells burden using BLI FI requires expression of gene-encoded fluorescent Image-specific metabolic pathways and protein protein or administration of tumor-selective expression with fluorescence imaging (FI) and fluorescence-tagged reporter probes to visualize fluorescent molecular probes metabolic pathway or enzyme activity High signal detection sensitivity of multiple fluorescent reporters within the same animal © All rights reserved. 2012 Inc. Nature America, Magnetic resonance imaging either T1 or T2 (or both) can be exploited to provide the contrast MRI is a powerful imaging tool for characterizing animal systems necessary to generate high-resolution MR images. and animal models of disease. In vivo MRI is a noninvasive and MRI signal intensity, which depends on the density of water nondestructive technique that enables a wide variety of longitudinal in soft tissue, has been well developed for the study of primary (time-course) studies that are not possible with other destructive brain39, prostate40 and liver tumors41, as well as for the evaluation of analytical methods. The vast majority of clinical and preclinical malignancies in many other organs and tissues. Lungs, however, MRI studies involve the detection of signal from hydrogen nuclei present unique challenges for MRI, which required the develop- in water. The observed signal depends on both the amount of water ment of new and innovative methods42–44. The unique challenges present in the sample and on the relaxation properties of that water.
Details
-
File Typepdf
-
Upload Time-
-
Content LanguagesEnglish
-
Upload UserAnonymous/Not logged-in
-
File Pages15 Page
-
File Size-